The rise of wearable technology has transformed how we monitor health, fitness, and even daily activities. Wearable devices, from smartwatches to fitness trackers, rely on analog circuits to process and interpret signals they receive from sensors. Although digital circuits often receive most of the attention, the role of analog circuits in wearable technology is equally critical. Analog circuits form the backbone of these devices. They are managing the analog signals that sensors capture and converting them into meaningful data. In this blog, we will explore the importance of analog circuits in wearable technology and discuss the essential components. We will delve into design challenges, and highlight key considerations for engineers looking to build next-generation wearable devices.
The Role of Analog Circuits in Wearable Devices
Analog circuits are integral to wearable technology due to their ability to handle real-world signals. Unlike digital circuits that deal with binary data, analog circuits process continuous signals. This is crucial for applications where data comes in various forms, such as temperature, pressure, sound, light, and bioelectrical signals. For instance, in a heart rate monitor, the sensor detects the electrical activity of the heart as an analog signal. Analog circuits then amplify, filter, and condition these signals before converting them into digital form for further processing.
In wearable technology, key analog functions include signal amplification, filtering, conditioning, data conversion, and power management. These functions ensure that the signals from sensors are correctly interpreted and that the device operates efficiently under varying conditions. Thus, understanding the role of analog circuits in these devices is vital for any engineer or designer working in the field of wearable technology.
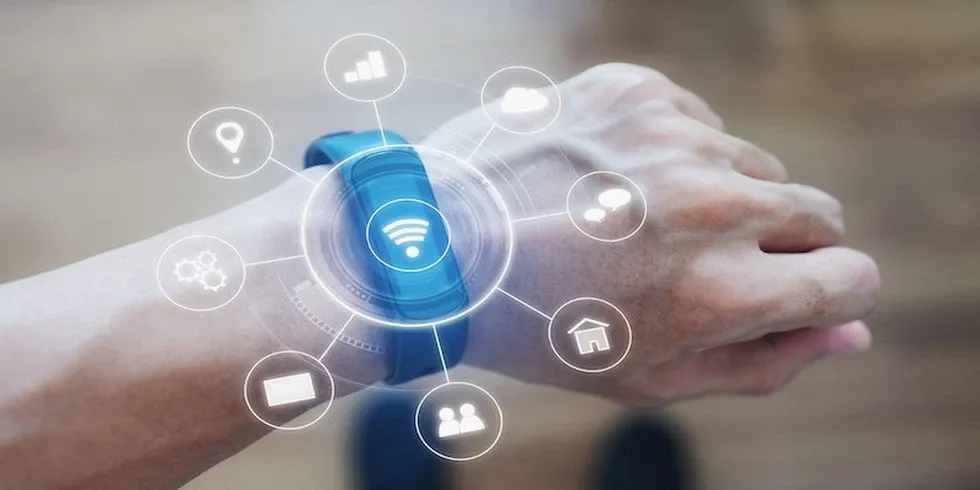
Key Components of Analog Circuits in Wearable Technology
To build effective analog circuits for wearable devices, engineers need to carefully select and design several core components. Each component plays a crucial role in ensuring the device’s overall performance, reliability, and power efficiency.
Operational Amplifiers (Op-Amps)
Operational amplifiers are fundamental building blocks in analog circuit design for wearable technology. They perform various tasks, such as signal amplification, filtering, and mathematical operations. In wearables, where space and power are limited, choosing low-power, low-noise op-amps with a high gain-bandwidth product is crucial. This choice ensures accurate signal processing without compromising battery life or adding excessive noise.
For example, in a wearable ECG monitor, op-amps amplify the tiny bioelectrical signals generated by the heart, making them suitable for further processing. The selection of an op-amp with a low input offset voltage, high common-mode rejection ratio (CMRR), and low bias current minimizes distortion and ensures signal integrity, which is vital for accurate heart rate monitoring.
Analog-to-Digital Converters (ADCs)
ADCs play a critical role in wearable devices by converting continuous analog signals into digital form for processing by the device’s microcontroller or digital signal processor (DSP). Choosing the right ADC involves balancing resolution, sampling rate, power consumption, and size. Higher resolution and faster sampling rates provide more accurate data, which is essential for medical-grade applications. However, these come at the cost of increased power consumption, which can impact battery life.
In applications like fitness trackers, low-power, 10- to 12-bit ADCs are often sufficient. However, in medical devices requiring high precision, such as glucose monitors or EEG devices, higher-resolution ADCs (16-bit or 24-bit) may be necessary to ensure accurate readings.
Sensors
Sensors are the primary interface between the wearable device and the external environment. They detect various parameters, such as heart rate, temperature, motion, and blood oxygen levels, and convert them into electrical signals. The choice of sensors affects the design of the entire analog front end (AFE) because different sensors have unique requirements in terms of signal conditioning, power supply, and interface compatibility.
For example, a photoplethysmography (PPG) sensor used in smartwatches to measure heart rate requires a low-noise, high-gain amplifier to detect the minute changes in light absorption due to blood flow. Similarly, a thermistor or thermocouple used for skin temperature measurement requires a precise, stable reference voltage and low-offset op-amps to ensure accurate readings.
Power Management Circuits
Power management is a critical aspect of wearable technology design. Wearable devices operate on small batteries that must last for days or even weeks without recharging. Analog power management circuits, such as low-dropout (LDO) regulators, DC-DC converters, and battery management ICs, ensure that the device operates efficiently across various power modes.
LDO regulators provide a stable output voltage with low noise. This is essential for powering sensitive analog components like sensors and op-amps. However, LDOs can be less efficient in power conversion, especially when the input voltage is significantly higher than the output voltage. In such cases, DC-DC converters are preferred, as they provide higher efficiency at the cost of increased circuit complexity and potential noise.
Filters
Filters are indispensable in analog circuit design for wearable technology, helping remove unwanted noise and interference from sensor signals. For example, a low-pass filter can remove high-frequency noise from an ECG signal, while a notch filter can eliminate power line interference at 50 or 60 Hz. Filters can be implemented using passive components (resistors, capacitors, inductors) or active components (op-amps).
Active filters are often preferred in wearable devices due to their ability to provide high gain, low noise, and greater control over frequency response. However, they consume more power than passive filters and require additional design considerations to ensure stability and minimize noise.
Advanced Noise Reduction Strategies
Noise is one of the primary challenges in designing analog circuits for wearables. Wearable devices operate in environments filled with various sources of electromagnetic interference (EMI), such as cell phones, Wi-Fi routers, and other electronic gadgets. Thus, reducing noise in analog circuits is crucial for maintaining signal integrity and ensuring accurate data acquisition.
Shielding and Grounding
Shielding and grounding are fundamental techniques for minimizing EMI and maintaining signal integrity. Proper shielding involves enclosing sensitive analog components in conductive materials that block external noise. Ground planes, on the other hand, provide a low-impedance path for return currents and reduce the effects of EMI. A well-designed ground plane also helps in minimizing common-mode noise, which can be particularly problematic in differential signal paths.
Differential Signaling
Differential signaling offers a robust solution to noise immunity issues in analog circuit design. Instead of sending a single-ended signal, differential signaling transmits two complementary signals along separate paths. This technique allows for the cancellation of common-mode noise because the interference affects both paths equally but in opposite phases. Differential signaling is particularly useful in applications like electrocardiography (ECG) or photoplethysmography (PPG) sensors, where small signal amplitudes make noise a significant concern.
Component Matching
Component matching involves selecting components with similar electrical characteristics to minimize discrepancies in circuit behavior. For example, using matched resistors in a differential amplifier can reduce the impact of common-mode noise. Similarly, matching capacitors can help stabilize filter circuits by ensuring that the cutoff frequencies remain consistent, which is critical for noise rejection.
Low-Power Design Techniques
Power efficiency is a cornerstone of wearable technology design. Wearable devices must operate for extended periods without frequent battery replacements or recharges. Therefore, designing low-power analog circuits is vital to meet user expectations and maintain the practicality of these devices.
Dynamic Power Scaling
Dynamic power scaling is a technique that adjusts the operating voltage and frequency of analog components based on the required performance level. For instance, when a wearable device is idle or in low-power mode, it reduces the supply voltage to analog circuits like amplifiers or converters, thereby lowering power consumption. Conversely, when high performance is needed, the device increases the supply voltage to ensure optimal circuit functionality. Implementing dynamic power scaling requires careful design considerations, including stable power management circuits and efficient control algorithms.
Ultra-Low Power Amplifiers
Selecting ultra-low-power operational amplifiers (op-amps) is another effective strategy for reducing power consumption in wearable devices. These specialized op-amps consume minimal current, often in the nanoampere (nA) range, while maintaining reasonable performance metrics like gain, bandwidth, and noise. Low-power op-amps are particularly useful in applications where signals are small and need amplification without drawing significant power, such as in wearable bio-sensors.
Energy Harvesting Circuits
Energy harvesting represents a cutting-edge approach to extending battery life in wearable devices. By converting ambient energy from sources like solar, thermal, or kinetic energy into electrical power, wearable devices can operate for longer periods without relying solely on batteries. Integrating energy harvesting circuits into the analog design requires careful consideration of factors like conversion efficiency, storage management, and power conditioning.
For example, a solar energy harvesting circuit might use a low-power boost converter to increase the voltage from a small solar panel to a usable level for the device. Simultaneously, the circuit must include energy storage elements, such as supercapacitors or rechargeable batteries, to store harvested energy and provide a stable power source when ambient energy is insufficient.
Integration of Analog and Digital Circuits
Modern wearable devices require a seamless integration of analog and digital circuits to maximize functionality and efficiency. This integration poses several challenges, particularly when it comes to maintaining signal integrity, minimizing power consumption, and ensuring reliable operation under varying conditions.
Mixed-Signal System-on-Chip (SoC) Solutions
Many wearable devices rely on mixed-signal SoCs that combine analog and digital components into a single chip. These SoCs integrate various functions, such as data acquisition, signal processing, communication, and power management, into a compact form factor. The key advantage of using mixed-signal SoCs is the reduction in size and power consumption compared to discrete solutions. Moreover, integrating analog and digital circuits on the same die helps minimize parasitic capacitance and inductance, leading to improved signal integrity and reduced noise.
Digital Calibration of Analog Circuits
Digital calibration techniques can significantly improve the performance of analog circuits in wearable devices. For instance, an integrated microcontroller can automatically adjust the offset and gain of an analog front end (AFE) based on calibration data stored in memory. This process compensates for component tolerances, temperature variations, and other factors that affect analog circuit performance. Digital calibration enhances the accuracy and reliability of analog circuits, making it particularly valuable for medical-grade wearable devices.
Digital Signal Processing (DSP) for Noise Reduction
DSP techniques can further enhance the performance of analog circuits by reducing noise and improving signal quality. For example, a wearable ECG monitor might use a DSP algorithm to filter out power line interference and baseline wander from the analog signal before further processing. DSP-based noise reduction complements traditional analog filtering techniques, allowing for more flexible and adaptive signal conditioning.
Thermal Management in Wearable Devices
Wearable devices are in constant contact with the human body, making thermal management a critical design consideration. Excessive heat generation can cause discomfort to users and degrade the performance of temperature-sensitive analog components. Therefore, effective thermal management strategies are essential to ensure both device reliability and user comfort.
Low-Power Thermal Sensors
Thermal sensors can monitor the device’s temperature and enable dynamic thermal management strategies. These sensors, often integrated into the analog circuitry, provide real-time feedback on the device’s thermal status. By monitoring temperature, wearable devices can dynamically adjust power consumption, clock speeds, or even shut down non-critical functions to prevent overheating.
Heat Dissipation Techniques
Designing for efficient heat dissipation involves selecting appropriate materials, component placement, and thermal interface materials (TIMs). Using materials with high thermal conductivity, such as aluminum or copper, helps dissipate heat away from critical components. Additionally, placing heat-generating components like power management ICs (PMICs) or microcontrollers away from sensitive analog circuits reduces the risk of thermal interference. Engineers can also use TIMs to enhance heat transfer between components and heat sinks or enclosures.
Low-Noise Analog Circuit Design
Noise reduction is fundamental in designing analog circuits for wearables. Since these devices often deal with small physiological signals, such as ECG or EEG, preserving signal integrity is crucial. Thus, advanced noise reduction techniques go beyond basic filtering and shielding.
Active Filtering Techniques
Active filtering using operational amplifiers offers a dynamic approach to noise reduction. For example, an active low-pass filter can remove high-frequency noise from an analog input signal before it reaches the ADC. Unlike passive filters, active filters can amplify weak signals, improving the overall signal-to-noise ratio (SNR). Carefully selecting low-noise op-amps and precise resistors and capacitors is vital to minimize noise generation within the filter circuit itself.
Chopper Stabilization
Chopper stabilization, or chopper-stabilized amplifiers, provides another technique for reducing low-frequency noise or offset drift. This method involves modulating the input signal to a higher frequency where the noise characteristics are more favorable, amplifying it, and then demodulating it back to the original frequency. This process effectively reduces flicker noise, which is prevalent in low-frequency applications like heart rate monitors and bio-potential sensors.
Bio-Compatible and Flexible Materials
Wearable devices must be comfortable and safe for extended periods. Incorporating bio-compatible and flexible materials into analog circuit design plays a significant role in achieving these goals. Additionally, using such materials ensures that the device adheres closely to the skin without causing irritation, making the device’s circuitry less obtrusive.
Conductive Polymers
Conductive polymers represent a class of materials that provide flexibility and bio-compatibility while maintaining electrical conductivity. Materials like PEDOT
(poly(3,4-ethylenedioxythiophene) polystyrene sulfonate) allow for stretchable circuits that conform to the human body’s contours. These materials are ideal for designing flexible interconnects and sensors in analog circuits, enabling the fabrication of stretchable and foldable devices.
Thin-Film Transistors (TFTs)
Thin-film transistors, constructed from materials like organic semiconductors or amorphous silicon, offer flexibility and low power consumption. These components are used to create flexible displays or sensors in wearables. TFTs, combined with flexible substrates, enable innovative circuit designs that can bend and stretch, providing a comfortable fit while maintaining functionality.
Advanced Signal Conditioning Techniques
Signal conditioning is the process of preparing an analog signal for conversion to digital form. For wearables, effective signal conditioning is essential to ensure accuracy and reliability, particularly when measuring biological signals prone to interference and variability.
Adaptive Filtering
Adaptive filtering dynamically adjusts its parameters based on the signal environment, making it highly effective for wearable devices operating in varying conditions. For example, an adaptive filter can be programmed to optimize its coefficients in real time, compensating for noise from movement or muscle contractions. This dynamic response enhances the reliability of wearable sensors, such as ECG monitors or electromyography (EMG) systems.
Analog Front End (AFE) Optimization
The analog front end (AFE) is critical in any wearable device’s signal chain. Optimizing the AFE design involves selecting the right combination of amplifiers, filters, and converters to ensure high accuracy, low noise, and low power consumption. AFEs designed specifically for bio-potential applications often incorporate instrumentation amplifiers with high input impedance and low offset voltage to accurately capture small biological signals.
Innovative Power Management Approaches
Power management is paramount in wearables, given their compact size and reliance on battery power. Innovating in power management involves not just reducing power consumption but also ensuring efficient energy storage and distribution throughout the device.
Power Gating
Power gating is an advanced technique where certain sections of the circuit are turned off when not in use. This method conserves energy by reducing leakage current, a common issue in miniaturized circuits. By designing circuits that can selectively shut down non-essential components during idle periods, wearables can achieve longer battery life without compromising performance.
Dynamic Voltage and Frequency Scaling (DVFS)
Dynamic Voltage and Frequency Scaling adjusts the voltage and frequency of the analog circuitry based on the required performance level. For example, during periods of low activity, the wearable device reduces both the operating voltage and frequency, conserving energy. Conversely, the device scales up performance in high-demand situations to maintain responsiveness. Implementing DVFS requires sophisticated power management ICs (PMICs) and a solid understanding of the device’s performance characteristics.
Energy Harvesting Integration
Integrating energy harvesting circuits into wearables offers a promising path to reducing dependency on battery power. Techniques such as thermoelectric energy harvesting can convert body heat into electrical energy, while piezoelectric materials capture energy from body movement. Efficiently combining these methods requires advanced analog circuitry that manages energy harvesting and storage seamlessly. Analog power conditioning circuits are vital to optimize the conversion and utilization of harvested energy.
Robust Analog Interfaces for Sensor Integration
Wearable devices often incorporate multiple sensors to monitor various parameters such as heart rate, temperature, and motion. Designing robust analog interfaces to integrate these sensors is critical to achieving reliable and accurate data acquisition.
Multiplexed Sensor Interfaces
A multiplexed sensor interface allows multiple sensors to share a single analog-to-digital converter (ADC) or amplifier. This will help in reducing the overall component count and power consumption. Proper multiplexing requires careful timing control and signal integrity management to ensure that signals from different sensors do not interfere with each other. Designing analog multiplexers with low on-resistance and minimal crosstalk is crucial for preserving signal quality.
Sensor Calibration and Compensation
Analog circuits can perform real-time sensor calibration and compensation to maintain accuracy. For instance, temperature sensors may experience drift due to environmental changes or aging. A dedicated analog calibration circuit can continuously adjust the sensor’s output to compensate for these effects, ensuring consistent performance. Moreover, analog offset and gain compensation circuits are often used in strain gauge-based sensors to eliminate errors caused by mechanical deformation or environmental factors.
Advanced Analog Circuit Topologies for Wearable Applications
Innovative circuit topologies are necessary to meet the unique challenges of wearable technology, such as miniaturization, power efficiency, and real-time data processing.
Current-Mode Circuits
Current-mode circuits offer several advantages over traditional voltage-mode circuits, including faster response times, lower power consumption, and reduced susceptibility to voltage noise. For example, current-mode analog-to-digital converters (ADCs) can achieve higher speeds and lower power consumption than their voltage-mode counterparts, making them ideal for applications like continuous health monitoring in wearables.
Switched-Capacitor Circuits
Switched-capacitor circuits provide another innovative approach, particularly in analog filtering and signal processing applications. By replacing resistors with capacitors and switches, these circuits offer precise control over cutoff frequencies and gain, with reduced size and power consumption. Switched-capacitor circuits are highly suitable for integrated AFEs in wearables, enabling compact, low-power designs that maintain high accuracy and reliability.
Future Prospects in Analog Circuit Design for Wearables
The future of wearable technology will undoubtedly involve continued innovation in analog circuit design. Several emerging trends are poised to shape the next generation of wearables, offering new possibilities for functionality, comfort, and user experience.
Hybrid Analog-Digital Circuits
Hybrid analog-digital circuits, which combine the strengths of both domains, will play a significant role in future wearables. For example, integrating analog machine learning algorithms with digital processing can enable real-time data analysis and decision-making, enhancing functionality while minimizing power consumption. Hybrid circuits can also provide more efficient sensor interfaces, combining analog pre-processing with digital post-processing to achieve optimal performance.
Printed and Flexible Electronics
Printed and flexible electronics will continue to gain prominence, allowing for more ergonomic and unobtrusive wearable devices. Innovations in materials science, such as conductive inks and flexible substrates, enable the design of analog circuits that conform to the body’s shape. These circuits can integrate seamlessly into clothing or be directly applied to the skin. This will create new avenues for continuous health monitoring and biometric analysis.
Ultra-Low Power Analog Design
As wearable technology continues to evolve, the demand for ultra-low-power analog circuit design will increase. Techniques such as sub-threshold operation, where circuits operate below the transistor threshold voltage, offer significant power savings. Additionally, leveraging advanced semiconductor processes, such as FD-SOI (Fully Depleted Silicon-On-Insulator) technology, can reduce power consumption and enhance performance in analog circuits.
Flexible and Stretchable Electronics
As wearable devices become more integrated with clothing and accessories, flexible and stretchable electronics are gaining traction. These new materials allow for the design of circuits that can bend, stretch, and conform to various shapes without losing functionality. Developing analog circuits with flexible substrates requires careful consideration of factors like mechanical stress, strain, and material fatigue. Engineers must also design circuits to accommodate changes in electrical properties, such as resistance or capacitance, caused by stretching or bending.
Ultra-Low Power Sensors and Interfaces
The demand for always-on, ultra-low-power operation is driving innovation in sensor technology and analog interfaces. Sensors that consume minimal power while maintaining high sensitivity and accuracy are crucial for applications like continuous health monitoring. Similarly, low-power analog interfaces, such as low-noise amplifiers, ADCs, and DACs, are essential for efficient data acquisition and signal processing. Advances in MEMS (Micro-Electro-Mechanical Systems) technology, for example, have led to the development of highly sensitive and low-power accelerometers, gyroscopes, and pressure sensors that are ideal for wearable devices.
Integrated Analog Artificial Intelligence (AI)
The integration of AI into analog circuits represents a cutting-edge trend that holds promise for wearable technology. Analog AI circuits, such as neuromorphic chips, can perform specific tasks, like pattern recognition or anomaly detection, with much lower power consumption than traditional digital processors. By incorporating analog AI circuits, wearable devices could offer advanced features, such as real-time health analytics or personalized feedback, without sacrificing battery life.
Design Challenges in Analog Circuits for Wearable Devices
Designing analog circuits for wearable devices presents unique challenges, given the constraints on size, power, and performance. Addressing these challenges requires a deep understanding of analog design principles and the specific requirements of wearable technology.
Power Efficiency
One of the most significant challenges in wearable technology is power efficiency. Wearable devices typically rely on small batteries with limited capacity, and users expect these devices to last for extended periods without frequent charging. Thus, every component in the analog circuit must consume minimal power while still meeting performance requirements.
For instance, engineers often face trade-offs between power consumption and performance when selecting op-amps, ADCs, and other analog components. Low-power components may offer reduced performance, such as lower bandwidth or higher noise, which could impact the accuracy and responsiveness of the device. Finding the right balance is crucial for achieving optimal power efficiency without compromising functionality.
Noise and Signal Integrity
Wearable devices operate in dynamic environments, often in close proximity to noise sources like smartphones, Wi-Fi routers, and other electronic devices. Maintaining signal integrity in such noisy environments is a critical challenge. Analog circuits must be designed to minimize noise and interference, which can degrade the performance of sensors and other components.
Shielding, proper grounding, and filtering techniques are essential for reducing noise and maintaining signal integrity. Engineers must also consider factors like component placement, PCB layout, and trace routing to minimize noise coupling and crosstalk. Using low-noise components and designing circuits with high common-mode rejection and low offset voltages can further enhance signal integrity.
Size Constraints
Wearable devices must be compact and lightweight, which imposes stringent size constraints on the analog circuits. Engineers need to select components that are small in size but still provide the required performance. Integrated circuits (ICs) that combine multiple functions, such as ADCs, amplifiers, and filters, into a single package can help reduce the overall footprint of the analog front end.
However, integrating multiple functions into a single IC can introduce additional design challenges, such as managing heat dissipation, minimizing crosstalk, and ensuring reliable operation under varying environmental conditions. Engineers must carefully evaluate these trade-offs when designing analog circuits for wearables.
Environmental Variability
Wearable devices are exposed to a wide range of environmental conditions, including temperature, humidity, motion, and pressure changes. Analog circuits must be robust enough to operate reliably under these varying conditions. For example, temperature changes can affect the performance of components like resistors, capacitors, and sensors, leading to drift and reduced accuracy.
To address this challenge, engineers often use temperature-compensated components, such as precision resistors with low temperature coefficients or thermistors for temperature sensing. Additionally, careful circuit design, calibration, and compensation techniques can help mitigate the effects of environmental variability and ensure consistent performance.
Key Considerations for Designing Analog Circuits in Wearable Technology
Designing analog circuits for wearable devices requires careful consideration of several factors to ensure optimal performance, reliability, and user satisfaction.
Component Selection
Selecting the right components is crucial for achieving the desired performance in wearable devices. Engineers must consider parameters such as power consumption, noise, size, and cost when choosing components. For example, low-power op-amps with high gain-bandwidth products are ideal for amplifying small sensor signals without introducing significant noise. Similarly, selecting ADCs with appropriate resolution and sampling rates ensures accurate data conversion while minimizing power consumption.
Circuit Design and Layout
Proper circuit design and layout are essential for minimizing noise, reducing power consumption, and ensuring reliable operation. Engineers must carefully route analog signals to avoid noise coupling and interference from digital components. Grounding and shielding techniques, such as star grounding and guard traces, can help minimize noise and improve signal integrity.
Additionally, component placement plays a significant role in analog circuit design. Placing sensitive analog components, like sensors and op-amps, away from noisy digital components and high-frequency circuits reduces the risk of interference. Using differential signal routing and balanced signal paths can further enhance noise immunity.
Power Management
Efficient power management is critical for wearable devices, as it directly impacts battery life and user experience. Engineers must design power management circuits that provide stable, noise-free power to sensitive analog components while minimizing energy consumption. Techniques such as dynamic voltage scaling, power gating, and adaptive power management can help optimize power usage based on the device’s operating conditions.
For example, using low-dropout (LDO) regulators with low quiescent current can provide stable power to analog circuits while minimizing power loss. In applications requiring higher efficiency, switching regulators like buck or boost converters can be used, but they must be carefully designed to minimize noise and ripple.
Testing and Calibration
Thorough testing and calibration are essential for ensuring the accuracy and reliability of analog circuits in wearable devices. Engineers must perform extensive testing under various operating conditions, such as temperature, humidity, and motion, to verify the circuit’s performance and identify potential issues. Calibration techniques, such as offset and gain correction, can help compensate for component tolerances and environmental variations.
Moreover, built-in self-test (BIST) and self-calibration features can enhance the reliability of wearable devices by enabling continuous monitoring and adjustment of circuit parameters. These features help maintain consistent performance over time and extend the device’s lifespan.
Conclusion
Analog circuits play a vital role in wearable technology, enabling accurate signal processing, efficient power management, and robust performance under varying conditions. As technology continues to advance, the importance of analog circuits in wearable devices will only grow. This will drive further innovation and enhancing the capabilities of these ubiquitous gadgets.
To have a better understanding of IoT Protocols, we recommend using Smowcode to boost your productivity and create great products 10x faster.
Try Smowcode for free and Boost your Productivity by 10x. : https://smowcode.com/
Do go through our other blogs to understand IoT concepts: https://blog.smowcode.com/smart-connectivity-wi-fi-in-the-iot-era/
Link to Modbus Blog: https://blog.smowcode.com/understanding-modbus-in-industrial-iot/