Wireless communication has become an integral part of modern technology. It enables a wide range of applications from consumer electronics to industrial automation. At the heart of these wireless communication systems lies a complex interplay of analog and digital circuits. They work together to transmit and receive signals efficiently and reliably. While digital design often gets the spotlight, analog circuit design is equally crucial. This is particularly in the context of wireless communication modules. In this blog, we will delve into the principles, challenges, and best practices involved in analog circuit design for wireless communication modules.
The Role of Analog Circuits in Wireless Communication
Analog circuits play a critical role in the functionality of wireless communication modules. These circuits are responsible for processing the continuous-time signals that carry the information through the airwaves. Unlike digital signals, which are represented by discrete values, analog signals vary continuously. This makes them ideal for representing the varying amplitudes, frequencies, and phases of radio waves.
In wireless communication modules, analog circuits perform several essential functions, including signal amplification, filtering, modulation, demodulation, and frequency synthesis. Each of these functions requires careful design. This ensures that the wireless module can transmit and receive signals with high fidelity, low noise, and minimal distortion. The performance of the analog circuits directly impacts the overall performance of the wireless communication system. This makes analog design a critical aspect of wireless module development.
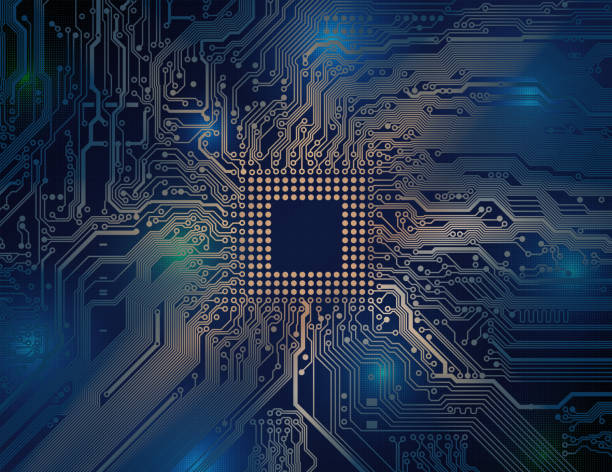
Signal Amplification: Boosting the Power of Wireless Signals
One of the primary tasks of analog circuits in wireless communication modules is signal amplification. Wireless signals, especially those transmitted over long distances or through obstacles, can weaken significantly before reaching the receiver. Amplifiers are used to boost these weak signals to a level that can be processed by the subsequent stages of the communication system.
However, signal amplification is not just about making the signal stronger; it’s about doing so without introducing excessive noise or distortion. The quality of the amplified signal is determined by several factors. Some of these factors are linearity of the amplifier, the noise figure, and the gain.
Linearity is crucial in wireless communication because non-linear amplification can lead to signal distortion, which can interfere with other channels and degrade the overall communication quality.
Noise figure, on the other hand, represents the amount of noise introduced by the amplifier itself. A low noise figure is desirable to preserve the signal-to-noise ratio (SNR) and ensure clear communication.
Finally, the gain of the amplifier determines the level of signal boost. However, excessive gain can lead to saturation, where the amplifier’s output reaches its maximum limit and can no longer increase with the input signal. This can cause clipping and further distortion.
In designing amplifiers for wireless communication modules, selecting the right type of amplifier is crucial. For instance, Low Noise Amplifiers (LNAs) are often used in the front-end of the receiver chain. They are important toamplify weak signals while introducing minimal noise. Power Amplifiers (PAs), on the other hand, are used in the transmitter chain. They boost the signal power to a level suitable for transmission over the air.
Filtering: Removing Unwanted Frequencies
Another critical function of analog circuits in wireless communication modules is filtering. Wireless signals are susceptible to interference from various sources, including other wireless devices, power lines, and even environmental noise. Filters are used to remove these unwanted frequencies. They ensure that only the desired signal reaches the next stage of the communication system.
There are several types of filters used in wireless communication, each with its specific purpose.
Low-pass filters
They allow signals below a certain frequency to pass through while attenuating higher frequencies. These are often used to remove high-frequency noise from the signal.
High-pass filters
These filters on the other hand, allow signals above a certain frequency to pass through while attenuating lower frequencies. These are useful for removing low-frequency noise and DC offsets.
Band-pass filters
Wireless communication systems frequently use these filters. They allow signals within a specific frequency range to pass through while attenuating signals outside this range. In wireless communication, where signals transmit over specific frequency bands, engineers must ensure that any interference outside these bands gets removed.
Band-stop filters
These filters, also known as notch filters, do the opposite of band-pass filters. They attenuate signals within a specific frequency range while allowing signals outside this range to pass through. These are useful for removing specific types of interference, such as narrowband noise or signals from other communication channels.
The design of filters for wireless communication modules requires careful consideration of several factors. This includes the desired frequency range, the level of attenuation required, and the quality factor (Q) of the filter. A high Q factor indicates a narrow bandwidth and sharp filtering. This is desirable in applications where precise frequency selection is required. However, high-Q filters can also be more difficult to design and may introduce more phase distortion. We have to check all parameters,which need to be managed carefully.
Modulation and Demodulation: Encoding and Decoding Information
Modulation is the process of encoding information onto a carrier signal for transmission over the air. This is a fundamental aspect of wireless communication. This approach allows multiple signals to be transmitted over the same frequency band without interfering with each other. Analog circuits play a critical role in both the modulation and demodulation processes.
Types of Modulation in Analog Circuits
In Amplitude Modulation (AM), the system encodes information by varying the amplitude of the carrier signal. Implementing AM is relatively simple, which makes it widely used in applications like radio broadcasting. However, noise and interference more easily affect AM, which can impact the quality of the received signal.
Frequency Modulation (FM) encodes information by varying the carrier signal’s frequency. FM reduces susceptibility to amplitude noise and provides better signal quality, making it ideal for FM radio and television broadcasting. However, FM requires a larger bandwidth than AM, which can limit its use in some applications.
Phase Modulation (PM) encodes information by varying the carrier signal’s phase. Engineers use PM in more advanced communication systems, such as digital modulation schemes like Phase-Shift Keying (PSK) and Quadrature Amplitude Modulation (QAM). These techniques offer higher data rates and better spectral efficiency, though they are more complex to implement.
Demodulation
Demodulation reverses the process of modulation by decoding the received signal to extract the original information. Analog circuits perform this decoding, often paired with digital signal processing (DSP) techniques to enhance the communication system’s performance.
Designing modulation and demodulation circuits for wireless communication modules demands a deep understanding of the specific modulation scheme and the characteristics of the wireless channel. Engineers must carefully consider factors such as signal-to-noise ratio (SNR), bandwidth efficiency, and error rates to ensure reliable communication.
Frequency Synthesis: Generating Stable Carrier Signals
Frequency synthesis is another critical function of analog circuits in wireless communication modules. A frequency synthesizer generates the stable carrier signals required for modulation and demodulation. These carrier signals must be highly stable and accurate. In these signals, any deviation can lead to errors in the transmitted or received information.
There are several methods for frequency synthesis, with the most common being Phase-Locked Loops (PLLs) and Direct Digital Synthesis (DDS). Each method has its advantages and is suited to different applications.
Phase-Locked Loops (PLLs)
Engineers widely use them in wireless communication systems because they generate highly stable frequencies over a wide range. A PLL locks the phase of a voltage-controlled oscillator (VCO) to the phase of a reference signal. By changing the division ratio in the feedback loop, engineers can adjust the output frequency, allowing for precise frequency control.
Engineers use PLLs in various applications, including generating local oscillator signals in receivers and providing clock signals for digital circuits. However, designing a PLL is complex and requires careful consideration of factors such as loop bandwidth, phase noise, and lock time. Additionally, the choice of VCO and the feedback loop’s design play a critical role in achieving the desired performance.
Direct Digital Synthesis (DDS)
Engineers use Direct Digital Synthesis (DDS) as another method of frequency synthesis that provides high resolution and fast frequency switching. DDS generates a waveform by accumulating phase information in a digital accumulator and converting it to an analog signal using a digital-to-analog converter (DAC). The system determines the output frequency based on how quickly the phase accumulator updates.
Engineers find DDS particularly useful in applications requiring precise frequency control and fast switching, such as in frequency-hopping communication systems. However, DDS systems can consume more power and be more complex than PLL-based systems, making them less suitable for some low-power applications.
The choice between PLL and DDS depends on the specific requirements of the wireless communication module, including the desired frequency range, resolution, switching speed, and power consumption. In some cases, engineers may use a combination of both methods to achieve the best performance.
The Importance of Analog Circuit Design in Wireless Communication
Analog circuits serve as the foundation of wireless communication, responsible for handling continuous signals that vary in amplitude, frequency, and phase. Unlike digital signals, which are discrete and binary, analog signals require precise manipulation to maintain their integrity throughout the transmission and reception processes.
Wireless communication modules rely on analog circuits to perform a myriad of functions, from signal conditioning and impedance matching to phase noise management and temperature compensation. Each of these tasks requires meticulous design to ensure that the wireless module can operate efficiently under varying conditions.
Signal Conditioning and Impedance Matching
Signal conditioning is one of the critical tasks that analog circuits must perform in wireless communication modules. The purpose of signal conditioning is to prepare the analog signal for further processing by amplifying, filtering, or otherwise modifying it to suit the requirements of the subsequent stages. This process is essential for maintaining the integrity of the signal, particularly when dealing with weak or noisy signals.
Impedance matching, another vital aspect of analog design, ensures that the maximum amount of signal power is transferred between different stages of the communication module. Mismatched impedances can lead to signal reflections, power loss, and reduced overall system performance. By carefully designing matching networks and selecting appropriate components, engineers can optimize signal transmission and reception, minimizing losses and maximizing efficiency.
Phase Noise Management in Analog Circuits
Phase noise, which refers to the short-term fluctuations in the phase of a signal, is a significant concern in wireless communication systems. High levels of phase noise can degrade the quality of the transmitted signal. This leads to increased bit error rates (BER) and reduced overall system performance.
Analog circuits play a crucial role in managing phase noise through the design of stable oscillators, low-noise amplifiers, and phase-locked loops (PLLs). By focusing on low phase noise designs, engineers can enhance the signal quality. They ensure that the wireless communication module operates effectively even in environments with significant interference or noise.
Temperature Compensation
Temperature variations can have a profound impact on the performance of analog circuits, particularly in wireless communication modules that operate in diverse environmental conditions. Components such as resistors, capacitors, and transistors exhibit temperature-dependent behaviors, which can lead to drift in the performance characteristics of the circuit.
Analog circuit designers must incorporate temperature compensation techniques. This ensures that the module operates consistently across a wide range of temperatures. This may involve selecting temperature-stable components, designing compensation networks, or employing feedback mechanisms. It is important to adjust circuit parameters in response to temperature changes.
Power Supply Design and Management
Power supply design is another critical aspect of analog circuit design for wireless communication modules. The power supply must deliver clean, stable voltage levels to the analog circuits, as fluctuations or noise in the power supply can significantly impact signal integrity.
Designing power supply circuits for wireless communication modules requires a deep understanding of the power requirements of the various analog components, as well as the ability to manage power efficiently, particularly in battery-operated devices. Engineers must design low-noise power supply circuits, incorporate voltage regulation techniques, and implement efficient power management strategies to ensure the module operates reliably and efficiently.
Advanced Techniques in Analog Circuit Design for Wireless Modules
As wireless communication technology continues to evolve, the demand for higher performance, lower power consumption, and greater integration has led to the development of advanced analog circuit design techniques. These techniques push the boundaries of what is possible, enabling wireless communication modules to meet the stringent requirements of modern applications.
High-Linearity Amplifiers
High-linearity amplifiers are essential in wireless communication modules, particularly in the transmitter and receiver chains. Linearity refers to the ability of an amplifier to accurately reproduce the input signal at its output without introducing significant distortion. In wireless communication, non-linearity can lead to signal distortion, intermodulation products, and spectral regrowth, all of which can degrade the quality of the transmitted signal and cause interference with adjacent channels.
To achieve high linearity, designers often employ techniques such as feedback, feedforward correction, and predistortion. Feedback reduces distortion by comparing the output signal with the input and making adjustments to minimize errors. Feedforward correction involves splitting the signal into two paths, amplifying the error signal, and then subtracting it from the main signal to cancel out distortion. Predistortion, on the other hand, introduces an inverse distortion to the input signal, so that the overall distortion at the output is minimized.
Low-Noise Design Practices
Minimizing noise is a top priority in analog circuit design for wireless communication modules. Noise can be introduced from various sources, including thermal noise, flicker noise, and phase noise. To design low-noise circuits, engineers must carefully select components, optimize circuit topologies, and implement noise-reduction techniques.
For instance, in low-noise amplifier (LNA) design, engineers often choose transistors with low noise figures, optimize the biasing conditions, and use matching networks to minimize the noise contribution. Additionally, shielding and proper layout techniques can help reduce noise coupling from external sources, further improving the signal-to-noise ratio (SNR).
Adaptive Analog Circuits
Adaptive analog circuits represent a cutting-edge approach to analog design, particularly in the context of wireless communication modules. These circuits dynamically adjust their parameters in response to changing conditions, such as variations in temperature, power supply voltage, or signal strength. By continuously monitoring the operating conditions and making real-time adjustments, adaptive circuits can maintain optimal performance even in challenging environments.
One example of an adaptive analog circuit is an adaptive gain amplifier, which adjusts its gain based on the input signal level. This allows the amplifier to provide sufficient gain for weak signals while avoiding saturation for strong signals. Adaptive filters, another example, can change their frequency response characteristics in real-time to reject unwanted signals or noise, ensuring that only the desired signal is processed.
Mixed-Signal Integration
Mixed-signal integration is a key trend in wireless communication module design, where analog and digital circuits are integrated onto the same chip or module. This integration offers several advantages, including reduced size, lower power consumption, and improved performance. However, it also presents significant challenges, particularly in managing the interactions between analog and digital circuits.
In mixed-signal design, engineers must carefully consider the layout and isolation of analog and digital components to minimize crosstalk and interference. Techniques such as guard rings, separate power and ground planes, and careful routing of high-speed digital signals can help mitigate these issues. Additionally, mixed-signal testing requires specialized techniques to ensure that both analog and digital circuits perform as expected.
High-Frequency Design Considerations
As wireless communication modules operate at increasingly higher frequencies, the design of analog circuits becomes more challenging. High-frequency design introduces issues such as parasitic inductance and capacitance, signal integrity problems, and electromagnetic interference (EMI).
To address these challenges, engineers must employ high-frequency design techniques, such as careful PCB layout, impedance control, and the use of low-loss materials. For instance, microstrip and stripline techniques can be used to create transmission lines with controlled impedance, minimizing signal reflections and losses. Additionally, the use of surface-mount components and short signal paths can help reduce parasitic effects, ensuring that the analog circuits perform optimally at high frequencies.
Future Trends in Analog Circuit Design for Wireless Communication
The field of wireless communication is continuously evolving, driven by the demand for faster data rates, lower power consumption, and greater connectivity. As a result, analog circuit design for wireless communication modules is also evolving, with several emerging trends shaping the future of the field.
Sub-THz and mmWave Design
The push for higher data rates and increased bandwidth is leading to the exploration of sub-THz and millimeter-wave (mmWave) frequencies for wireless communication. These frequencies offer significant advantages in terms of bandwidth availability and data throughput. They also present new challenges for analog circuit design.
Designing analog circuits for sub-THz and mmWave frequencies requires a deep understanding of high-frequency behavior, including the effects of parasitics, skin effect, and dielectric losses. Additionally, the design of components such as amplifiers, mixers, and oscillators becomes more complex at these frequencies. This design requires innovative solutions to achieve the desired performance.
RF and Analog ICs for 5G and Beyond
The rollout of 5G networks and the development of future wireless communication standards are driving the demand for advanced RF and analog integrated circuits (ICs). These ICs must support a wide range of frequencies, including the traditional sub-6 GHz bands and the new mmWave bands used in 5G.
RF and analog IC design for 5G involves challenges such as wideband operation, linearity, and power efficiency. Additionally, the integration of multiple functions, such as amplification, mixing, and filtering, onto a single chip requires careful consideration of interactions between different circuit blocks. As 5G evolves and new standards such as 6G are developed, the demand for innovative RF and analog IC designs will only increase.
Power-Efficient Analog Design for IoT and Wearables
The proliferation of IoT devices and wearable technology is driving the need for power-efficient analog circuit design. These devices often operate on limited power sources, such as batteries or energy harvesting, making power efficiency a top priority.
Analog designers must focus on reducing power consumption without compromising performance. This may involve designing circuits with low quiescent current, using power-gating techniques to disable unused circuit blocks, and optimizing biasing conditions for low power operation. As the IoT and wearable markets continue to grow, the demand for power-efficient analog designs will remain strong.
Artificial Intelligence and Machine Learning in Analog Design
Artificial intelligence (AI) and machine learning (ML) are beginning to make their way into the field of analog circuit design. These technologies offer the potential to revolutionize the design process by automating tasks such as circuit optimization, parameter tuning, and fault detection.
AI and ML algorithms can analyze vast amounts of design data to identify patterns and make predictions, helping designers achieve optimal performance with less manual effort. Additionally, AI and ML can be used in adaptive analog circuits. We can continuously optimize performance in real-time, based on changing conditions.
Challenges in Analog Circuit Design for Wireless Communication
Designing analog circuits for wireless communication modules is a complex and challenging task that requires a deep understanding of both the theoretical principles and practical considerations involved. Several challenges must be addressed to achieve optimal performance, reliability, and efficiency.
Dealing with Practical Analog Values
Engineers face a primary challenge in analog design: dealing with non-idealities in components and circuits. Unlike digital circuits, which represent signals with discrete values, analog circuits must handle the continuous nature of electrical signals. These signals are susceptible to noise, distortion, and other non-idealities that can arise from various sources, including component tolerances, temperature variations, power supply fluctuations, and parasitic effects.
To address these challenges, analog designers must carefully select components and design circuits with robust margins. They need to implement techniques to minimize the impact of non-idealities. For example, engineers use feedback to stabilize circuits and reduce the effects of component variations. They also employ matching techniques to ensure that critical components perform consistently across different conditions.
Power Consumption
Another challenge is power consumption. Wireless communication modules are often used in battery-powered devices, where power efficiency is critical. Analog circuits, particularly amplifiers and frequency synthesizers, can consume significant power. Optimizing their efficiency is essential to prolong battery life and reduce heat dissipation.
Power-efficient design techniques, such as biasing for low quiescent current, using low-power components, and optimizing circuit topologies, are essential for reducing power consumption. Additionally, designers must consider the trade-offs between power efficiency and performance, as more efficient circuits may not always provide the best signal quality or bandwidth.
Integration is another challenge in analog design for wireless communication modules. As devices become smaller and more integrated, analog circuits must be designed to coexist with digital circuits on the same chip. This can lead to issues such as crosstalk, interference, and layout constraints. This degrades the performance of the analog circuits.
To address these challenges, analog designers must work closely with digital designers. They have to ensure that the layout, power supply, and grounding schemes are optimized for both analog and digital circuits. Techniques such as isolation, shielding, and careful layout planning can help minimize the impact of integration on analog performance.
Best Practices for Analog Circuit Design in Wireless Communication Modules
Given the challenges involved in analog circuit design for wireless communication modules, following best practices is essential to achieving optimal performance and reliability. Here are some key best practices to consider:
Understand the System Requirements
Before starting the design, it’s essential to have a clear understanding of the system requirements, including the frequency range, signal bandwidth, power consumption, and noise tolerance. This understanding will guide the selection of components, circuit topologies, and design techniques.
Simulate and Model
Use simulation tools to model the analog circuits and identify potential issues before prototyping. Simulations can help optimize the design, identify non-idealities, and validate the performance under different conditions. However, be aware of the limitations of simulations and complement them with real-world testing.
Optimize for Noise Performance
Noise is a critical factor in wireless communication, and minimizing it is essential for achieving high signal quality. Use techniques such as low-noise amplifiers, proper grounding, and shielding to reduce noise. Additionally, consider the trade-offs between noise performance and other factors such as power consumption and linearity.
Consider Power Efficiency
Power efficiency is crucial in wireless communication modules, especially for battery-powered devices. Use low-power components, optimize biasing, and design circuits for low quiescent current to minimize power consumption. Additionally, consider the impact of power efficiency on other performance parameters and balance the trade-offs accordingly.
Design for Integration of Analog Circuits
If the analog circuits are to be integrated with digital circuits on the same chip, consider the impact of integration on performance. Use techniques such as isolation, shielding, and careful layout planning to minimize crosstalk and interference. The industry must work with digital designers to ensure that the layout and power supply schemes are optimized for analog and digital circuits.
Prototype and Test your Analog Circuits
Once the design is complete, build prototypes and test them under real-world conditions. Testing will help identify any issues that were not apparent during simulation and allow for fine-tuning of the design. Additionally, use testing to validate the performance against the system requirements and ensure that the design meets the desired specifications.
Conclusion
Analog circuit design is a critical aspect of wireless communication modules, playing a vital role in signal amplification, filtering, modulation, demodulation, and frequency synthesis. Despite the challenges involved, following best practices and carefully considering the system requirements can lead to the successful design of high-performance analog circuits for wireless communication.
As wireless communication technology continues to evolve, the demand for more efficient, reliable, and integrated analog circuits will only grow. By staying informed about the latest design techniques and tools, analog designers can continue to push the boundaries of what’s possible in wireless communication and contribute to the development of the next generation of wireless devices.
To have a better understanding of IoT Protocols and microelectronics, we recommend using Smowcode to boost your productivity and create great products 10x faster.
Try Smowcode for free and Boost your Productivity by 10x. : https://smowcode.com/
Do go through our other blogs to understand IoT concepts: https://blog.smowcode.com/smart-connectivity-wi-fi-in-the-iot-era/
Link to Modbus Blog: https://blog.smowcode.com/understanding-modbus-in-industrial-iot/