In the world of embedded systems, amplifiers are crucial components that ensure accurate signal processing. This makes them indispensable in applications ranging from audio systems to sensor interfaces. Among the various types of amplifiers, low distortion amplifiers stand out for their ability to preserve the integrity of signals. They minimize unwanted alterations. Distortion in amplifiers can lead to inaccuracies, noise, and a host of other issues. This compromises with the performance of an embedded system.
Understanding Distortion in Amplifiers
Amplifiers are designed to increase the amplitude of signals without altering their original characteristics. However, real-world amplifiers are not perfect. They often introduce various forms of distortion, which can significantly degrade the quality and accuracy of the output signal. Distortion occurs when an amplifier alters the original signal in any way, deviating from its intended linear operation. The primary types of distortion that impact signal integrity include harmonic distortion, intermodulation distortion, and phase distortion. Each of these introduces specific errors into the signal, which can have detrimental effects depending on the application.
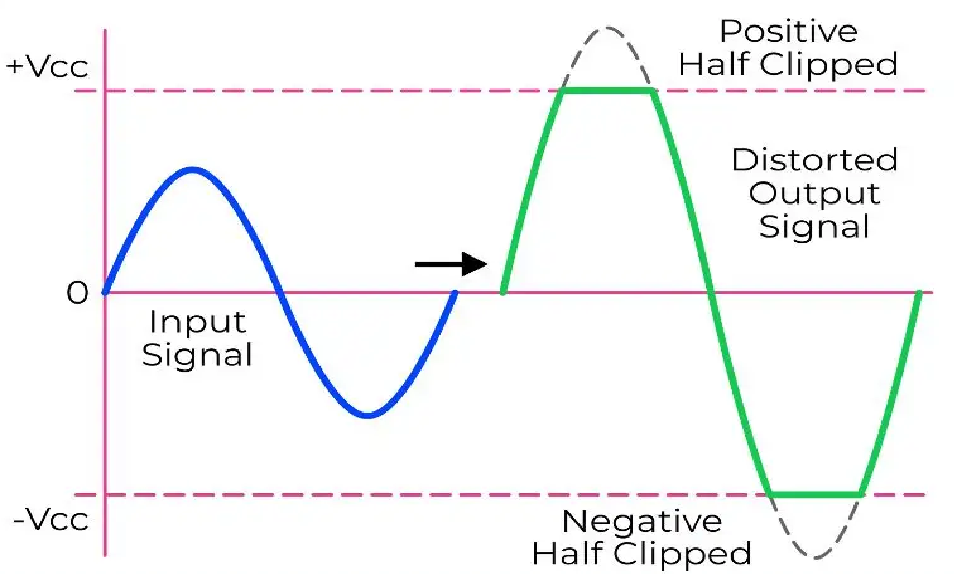
Harmonic Distortion
Harmonic distortion is one of the most common forms of distortion in amplifiers. It occurs when the amplifier generates harmonics of the input signal’s fundamental frequency. These harmonics are unwanted and can lead to a signal that differs significantly from the original. Theseunwanted harmonics affect applications where signal fidelity is crucial. For example, audio systems, communication systems, and measurement instruments.
Origin of Harmonic Distortion
Harmonic distortion arises from the non-linear behavior of the amplifier. In an ideal amplifier, the output is a perfect, scaled replica of the input signal. However, in reality, no amplifier is perfectly linear. When an amplifier operates in its non-linear region, it amplifies the fundamental frequency of the input signal. It also creates multiples of this frequency, known as harmonics. For example, if the input signal has a frequency of 1 kHz, the amplifier might generate additional frequencies at 2 kHz, 3 kHz, and so on. These additional frequencies are not present in the original signal and are considered distortions.
Impact on Signal Quality
Harmonic distortion significantly impacts the quality of the amplified signal. In audio systems, for instance, harmonic distortion can manifest as a harsh or “muddy” sound. In these signals, we have to comprimise the purity of the original audio. This type of distortion is especially noticeable in high-fidelity audio systems. In these systems, even a small amount of harmonic distortion can degrade the listening experience. The human ear is particularly sensitive to harmonics that are not musically related to the original signal. This leads to unpleasant artifacts in the sound.
Measuring Harmonic Distortion
Engineers often use the Total Harmonic Distortion (THD) metric to quantify harmonic distortion. THD measures the ratio of the sum of the powers of all harmonic components to the power of the fundamental frequency. A lower THD indicates that the amplifier introduces less harmonic distortion, which is essential in high-precision applications. For professional audio equipment, engineers typically require a THD of less than 0.1% to ensure high sound quality.
Mitigating Harmonic Distortion
To minimize harmonic distortion, designers can employ several strategies. They can operate the amplifier within its linear range, use feedback mechanisms to stabilize the amplifier’s performance, and select high-quality components that exhibit minimal non-linearity. In digital systems, engineers can also use signal processing techniques like harmonic cancellation to reduce the impact of harmonic distortion on the output signal.
Intermodulation Distortion
Intermodulation distortion (IMD) occurs when an amplifier processes two or more signals simultaneously, leading to the creation of new frequencies that were not present in the original signals. These new frequencies, known as intermodulation products, are often the sum or difference of the original frequencies and their harmonics. IMD is particularly problematic in communication systems and multi-channel audio systems, where it can interfere with the desired signal and degrade overall system performance.
Origin of Intermodulation Distortion
IMD arises from the non-linear mixing of multiple input signals within the amplifier. When two or more signals pass through a non-linear system, the non-linearity causes the signals to interact with each other, generating new frequency components. For example, if two signals with frequencies of 1 kHz and 1.5 kHz are input to an amplifier, IMD may generate additional frequencies at 500 Hz (difference frequency) and 2.5 kHz (sum frequency). These intermodulation products are not harmonically related to the original signals and can occur within the bandwidth of the desired signals, making them particularly troublesome.
Impact on Signal Quality
In communication systems, IMD can cause significant interference, leading to poor signal clarity and reduced data transmission rates. This is especially critical in radio frequency (RF) applications, where the presence of intermodulation products can lead to cross-talk between channels, making it difficult to distinguish between the intended signals. In audio systems, IMD can create dissonant tones that are not harmonically related to the original audio signals. This leads to a distortion that is unpleasant to the ear.
Measuring Intermodulation Distortion
IMD is often measured using the IMD ratio, which compares the amplitude of the intermodulation products to the amplitude of the original signals. A lower IMD ratio indicates that the amplifier introduces less intermodulation distortion. In RF applications, the third-order intermodulation (IM3) product is particularly important, as it often falls within the frequency band of the desired signal, making it more likely to interfere with the signal.
Mitigating Intermodulation Distortion
To reduce IMD, designers can use amplifiers with a high linearity. They ensure that the amplifier operates well within its linear region even when processing multiple signals. Additionally, careful filtering of the input signals can help to remove unwanted frequency components that could interact and produce intermodulation products. In multi-channel systems, isolating the channels and using separate amplification paths for each signal can also reduce the likelihood of IMD.
Phase Distortion
Phase distortion occurs when different frequency components of a signal experience varying delays as they pass through the amplifier. This results in a phase shift that can cause the output signal to become out of phase with the original. Phase distortion is particularly problematic in applications requiring precise timing, such as digital communication systems, audio systems, and control systems. In these systems, even small phase errors can lead to significant performance degradation.
Origin of Phase Distortion
Phase distortion arises from the frequency-dependent nature of the amplifier’s response. In an ideal amplifier, all frequency components of the input signal would experience the same delay. This preserves the phase relationships between them. However, in real-world amplifiers, certain frequencies may be delayed more than others due to factors such as parasitic capacitance, inductance, or the design of the amplifier’s feedback network. This frequency-dependent delay leads to phase distortion, where the output signal’s phase is altered relative to the input signal.
Impact on Signal Quality
Phase distortion can have a profound impact on systems that rely on precise timing. In digital communication systems, for instance, phase distortion can lead to bit errors, as the timing of the signal edges becomes misaligned, making it difficult to accurately decode the transmitted data. In audio systems, phase distortion can result in a loss of clarity and spatial imaging, as the phase relationships between different frequencies are altered. This can make instruments or voices sound “blurry” or “smeared,” reducing the overall quality of the sound.
Measuring Phase Distortion
Phase distortion is often measured using phase shift metrics. Thes metrics quantify the difference in phase between the input and output signals across different frequencies. Another common measurement is group delay. It represents the derivative of the phase shift with respect to frequency.It indicates how much delay a signal experiences as a function of frequency. A lower group delay variation across the frequency band of interest is desirable, as it indicates less phase distortion.
Mitigating Phase Distortion
Designers can minimize phase distortion by carefully designing the amplifier’s frequency response to be as flat as possible across the desired frequency range. Using phase compensation techniques, such as adding all-pass filters, can also help to equalize the phase response and reduce distortion. In digital systems, pre-compensation techniques can be employed. In these techniques,the signal is pre-distorted in a way that counteracts the expected phase distortion introduced by the amplifier.
The Importance of Low Distortion Amplifiers in Embedded Systems
Embedded systems require a high degree of signal fidelity, especially in environments where maintaining signal integrity is crucial. Whether handling audio processing, sensor data acquisition, or communication systems, engineers must ensure that the output signal closely matches the input. To achieve this, they use low distortion amplifiers specifically designed to minimize distortions.
Audio Processing
In audio systems, preserving sound quality is essential. Even minor distortions can introduce audible artifacts, degrading the listening experience. Engineers use low distortion amplifiers in preamplifiers, power amplifiers, and headphone amplifiers to maintain the clarity and authenticity of the audio signal, ensuring it remains true to the original recording.
Sensor Interfaces
Sensors in embedded systems often generate weak analog signals that require amplification before microcontrollers can process them. Engineers rely on low distortion amplifiers to boost these signals without introducing errors, allowing for precise measurement and processing.
Communication Systems
In communication systems, maintaining signal integrity is critical for reliable data transmission. Engineers use low distortion amplifiers to ensure the signal remains clear as it is transmitted and received. Thus they reduce data errors and enhancing overall system performance.
Key Design Considerations for Low Distortion Amplifiers
Designing a low distortion amplifier involves careful consideration of several factors that can influence performance. These include the choice of components, circuit topology, feedback mechanisms, and power supply design.
Component Selection
The choice of transistors, resistors, capacitors, and other components plays a significant role in determining the level of distortion in an amplifier. High-quality, low-noise components are essential for minimizing distortion. For instance, using precision resistors with tight tolerance values can reduce variations in the amplifier’s performance, thereby reducing distortion.
Circuit Topology
The design of the amplifier circuit itself is crucial. Common topologies for low distortion amplifiers include differential amplifiers, class A amplifiers, and fully complementary push-pull amplifiers. Each topology has its own advantages and trade-offs in terms of distortion, efficiency, and complexity.
- Differential Amplifiers: These amplifiers use two input signals of opposite phases to reduce common-mode noise and distortion. This topology is particularly effective in reducing harmonic distortion.
- Class A Amplifiers: Class A amplifiers operate with all transistors conducting for the entire input signal cycle, providing excellent linearity and low distortion at the cost of efficiency.
- Fully Complementary Push-Pull Amplifiers: This topology uses pairs of transistors that alternately conduct during each half of the signal cycle, reducing crossover distortion and improving overall linearity.
Feedback Mechanisms
Negative feedback reduces distortion in amplifiers by feeding a portion of the output signal back to the input. This technique linearizes the amplifier’s response, effectively lowering harmonic distortion and enhancing overall stability. Engineers must carefully optimize the design of the feedback network to prevent phase shifts that could cause instability or oscillations.
Power Supply Design
Power supply design is another crucial element in low distortion amplifier design. Engineers use stable, low-noise power supplies to minimize power supply-induced distortion. They often incorporate linear regulators to provide clean, stable voltage rails, ensuring the amplifier’s performance remains unaffected by power supply fluctuations. Additionally, engineers use decoupling capacitors to filter out high-frequency noise, preventing it from coupling into the amplifier’s signal path and degrading signal quality.
Practical Applications of Low Distortion Amplifiers in Embedded Systems
Low distortion amplifiers play a vital role in ensuring signal fidelity in embedded systems, where precision and accuracy are critical. The ability of these amplifiers to preserve the integrity of signals makes them indispensable across various applications.
High-Fidelity Audio Systems
High-fidelity audio systems demand an unwavering commitment to sound quality. In these systems, low distortion amplifiers are essential for preserving the audio signal’s purity. They ensure that the output remains faithful to the original recording. This requirement is particularly significant in applications such as studio monitors, audiophile-grade amplifiers, and high-quality headphone amplifiers.
Studio Monitors
Studio monitors require precise and accurate sound reproduction for professional audio work, such as mixing and mastering. Engineers rely on low distortion amplifiers to ensure that what they hear through the monitors is an accurate representation of the recorded material. Any distortion introduced by the amplifier can lead to erroneous adjustments in the mixing process, potentially compromising the final product. Low distortion amplifiers maintain the integrity of the signal, allowing audio professionals to make informed decisions.
Audiophile-Grade Amplifiers
Audiophiles are individuals who seek the highest quality sound reproduction, often investing in premium audio equipment. Low distortion amplifiers are a cornerstone of audiophile-grade systems, as they minimize harmonic distortion and intermodulation, two types of distortions that can alter the sound’s natural quality. By preserving the nuances of the original recording, low distortion amplifiers provide a listening experience that is as close as possible to a live performance.
High-Quality Headphone Amplifiers
Headphone amplifiers drive headphones with sufficient power while preserving audio fidelity. Low distortion is crucial in these amplifiers because high-quality headphones can reveal even the slightest imperfections in the sound. The proximity of the headphones to the ear makes detecting minor distortions easier. Therefore, engineers use low distortion amplifiers to ensure that the sound delivered to the listener remains clear, detailed, and free from unwanted artifacts.
Digital-to-Analog Conversion in Audio Systems
In high-fidelity audio systems, digital sources such as CDs, streaming services, or digital audio workstations (DAWs) require digital-to-analog converters (DACs) to convert digital audio signals into analog form for playback. Engineers often use low distortion amplifiers in the analog stage following the DAC. These amplifiers ensure that the conversion process does not introduce any unwanted distortion, preserving the quality of the audio signal throughout the entire playback chain, from the digital source to the listener’s ears.
Home Theater Systems
In home theater systems, engineers use low distortion amplifiers to enhance the audio experience by providing clear, powerful sound reproduction. These amplifiers are particularly important in multi-channel systems, where maintaining signal fidelity across all channels is crucial for creating an immersive audio environment. Whether ensuring dialogue clarity or accurately reproducing sound effects, low distortion amplifiers play a key role in delivering high-quality audio.
Precision Measurement Systems
Precision measurement systems rely on accurate signal amplification to ensure the integrity of the data collected from sensors and transducers. Low distortion amplifiers are crucial in these systems, as they minimize errors and noise that could compromise the accuracy of the measurements. These amplifiers find applications in scientific instruments, medical devices, and industrial control systems, where precision is paramount.
Scientific Instruments
In scientific research, instruments like oscilloscopes, spectrum analyzers, and data acquisition systems measure and analyze various physical phenomena. Researchers rely on low distortion amplifiers in these instruments to ensure that the signals they measure remain unaltered and free from distortion. For example, in an oscilloscope, low distortion amplifiers ensure that the waveform displayed on the screen accurately represents the input signal. This level of accuracy is crucial for researchers who need precise measurements to draw valid conclusions and make significant discoveries.
Medical Devices
In medical devices such as electrocardiographs (ECGs), electroencephalographs (EEGs), and blood pressure monitors, accurate amplification of biological signals is essential. These signals are often weak and susceptible to interference from noise and other sources. Engineers use low distortion amplifiers to amplify these signals while preserving their integrity, which allows for accurate diagnosis and monitoring of patients’ conditions. In critical applications like these, even minor distortions can lead to incorrect diagnoses or missed abnormalities, making the use of low distortion amplifiers indispensable.
Industrial Control Systems
Industrial control systems often rely on sensors to monitor and control processes. These sensors generate analog signals that must be accurately amplified before being processed by the control system. Low distortion amplifiers ensure that the amplified signals accurately reflect the monitored conditions. For example, in a temperature control system, a low distortion amplifier accurately amplifies the signal from the temperature sensor, allowing the control system to maintain the desired temperature with precision. Any distortion in the signal could result in incorrect temperature readings and, consequently, improper control actions.
Environmental Monitoring Systems
Environmental monitoring systems measure parameters such as temperature, humidity, air quality, and radiation levels. These systems often rely on low distortion amplifiers to accurately amplify signals from sensors that detect these parameters. For example, in air quality monitoring, low distortion amplifiers ensure that the signals from gas sensors are accurately amplified, allowing for precise measurement of pollutant concentrations. Accurate environmental monitoring is crucial for assessing and mitigating the impact of environmental hazards on public health and safety.
Calibration and Test Equipment
Calibration and test equipment are used to ensure that instruments and sensors provide accurate measurements. Low distortion amplifiers are often used in these systems to generate precise reference signals for calibration purposes. By minimizing distortion, these amplifiers ensure that the calibration process is accurate, allowing instruments to provide reliable measurements in their respective applications.
Communication Systems
Communication systems require the transmission and reception of signals with high fidelity to ensure reliable data transfer. Low distortion amplifiers play a critical role in maintaining the integrity of these signals. They are used particularly in radio frequency (RF) and wireless communication systems. In these systems,signal distortion can lead to data loss, communication errors, and reduced system performance.
RF Communication Systems
In RF communication systems, engineers transmit and receive signals at high frequencies. They use low distortion amplifiers to amplify these signals without introducing unwanted harmonics or intermodulation products. These unwanted harmonics and noise can interfere with adjacent channels. This practice is crucial in applications such as cellular networks, satellite communications, and broadcasting, where maintaining signal integrity ensures reliable communication. For example, in a cellular base station, engineers use low distortion amplifiers to keep the transmitted RF signal clean and free from distortion. This approach reduces the likelihood of interference with neighboring channels and improves the overall quality of service.
Satellite Communication
Satellite communication systems rely on the transmission of signals over long distances, often through the Earth’s atmosphere. There is a high probability that signal attenuation and distortion can occur. Low distortion amplifiers are used in both the uplink and downlink paths. They ensure that the signals are transmitted and received with minimal distortion. This is crucial for maintaining the quality and reliability of satellite communications, whether for television broadcasting, internet services, or military applications. By preserving the integrity of the signal, low distortion amplifiers help ensure that the data transmitted via satellite is received accurately on the ground.
Wireless Communication
Wireless communication systems, such as Wi-Fi, Bluetooth, and Zigbee, require low distortion amplifiers to maintain signal quality during transmission and reception. In these systems, low distortion amplifiers are used to amplify the RF signals generated by the transmitter and received by the receiver. This amplification must be done with minimal distortion to ensure that the transmitted data is accurately received and decoded. For example, in a Wi-Fi router, low distortion amplifiers ensure that the transmitted signal is strong enough to cover the desired area. This strength should hold even when introducing errors that could disrupt the connection. Similarly, in a Bluetooth device, low distortion amplifiers help maintain a stable and reliable connection by preserving the integrity of the transmitted signal.
Radar Systems
Radar systems use radio waves to detect and locate objects, such as aircraft, ships, and vehicles. Low distortion amplifiers are used in radar transmitters and receivers to ensure that the transmitted and received signals remain accurate and free from distortion. In the transmitter, low distortion amplifiers ensure that the radar signal is transmitted with high fidelity, allowing for accurate detection of targets. In the receiver, these amplifiers help amplify the weak return signals without introducing noise or distortion, enabling precise measurement of the target’s range, speed, and position. The use of low distortion amplifiers in radar systems is critical for applications such as air traffic control, defense, and automotive safety systems.
Optical Communication Systems
Optical communication systems use light waves to transmit data over fiber optic cables. In these systems, low distortion amplifiers are used to amplify the optical signals without introducing noise or distortion. This is particularly important in long-distance fiber optic communication, where signal attenuation can occur over the length of the cable. Low distortion amplifiers help maintain the quality of the optical signal, ensuring that data is transmitted accurately over long distances. By preserving the integrity of the signal, these amplifiers enable high-speed, high-capacity data transmission in optical communication networks.
Instrumentation Amplifiers
Instrumentation amplifiers are specialized amplifiers designed to amplify small differential signals while rejecting common-mode noise. Low distortion instrumentation amplifiers are essential in applications where signal accuracy is paramount, such as medical devices, strain gauge sensors, and other precision measurement systems.
Medical Devices
In medical devices, instrumentation amplifiers are used to amplify small bioelectric signals. They signals are like those generated by the heart (ECG) or brain (EEG). These signals are often weak and susceptible to interference from noise and other sources. Low distortion instrumentation amplifiers ensure that the amplified signal accurately represents the original bioelectric signal. This allows for accurate diagnosis and monitoring of patients’ conditions. For example, in an ECG machine, a low distortion instrumentation amplifier ensures that the amplified heart signal is clear and free from noise. This enables healthcare professionals to accurately assess the patient’s heart condition.
Strain Gauge Sensors
Strain gauge sensors measure the deformation (strain) of an object under load. These sensors produce small differential signals that need accurate amplification to obtain precise measurements. Engineers use low distortion instrumentation amplifiers to amplify the signals from strain gauges while rejecting common-mode noise. These amplifiers ensure that the measurements accurately reflect the strain on the object. This accuracy is particularly crucial in applications like structural health monitoring, where precise strain measurements assess the integrity of bridges, buildings, and other structures.
Bridge Circuits
Bridge circuits, such as Wheatstone bridges, measure physical quantities like temperature, pressure, and force using sensors. Engineers use these circuits to produce small differential signals that require accurate amplification. Low distortion instrumentation amplifiers amplify the output of bridge circuits, ensuring that the amplified signal precisely represents the physical quantity being measured. For example, in a pressure sensor, engineers use a low distortion instrumentation amplifier to accurately reflect the pressure applied to the sensor, enabling precise measurement and control in industrial processes.
Precision Data Acquisition
In precision data acquisition systems, accurate measurement and recording of analog signals are critical. Engineers use low distortion instrumentation amplifiers to amplify the analog signals before digitizing them with an analog-to-digital converter (ADC). By minimizing distortion, these amplifiers ensure that the digitized signal accurately represents the original analog signal, allowing for precise data analysis and interpretation. Precision data acquisition systems are essential in applications such as scientific research, industrial automation, and environmental monitoring.
Temperature Measurement Systems
Temperature measurement systems often use thermocouples or resistance temperature detectors (RTDs) to measure temperature. These sensors produce small differential signals that require accurate amplification. Low distortion instrumentation amplifiers are used to amplify the signals from temperature sensors, ensuring that the amplified signal accurately reflects the temperature being measured. This is particularly important in applications such as process control, where accurate temperature measurements are essential for maintaining product quality and safety.
Sensor Signal Conditioning
In embedded systems that interface with sensors, low distortion amplifiers are used to condition the sensor signals before they are converted to digital form by an analog-to-digital converter (ADC). This ensures that the sensor data is accurate and reliable, allowing the microcontroller to make informed decisions based on the processed signals.
Temperature Sensors
Temperature sensors like thermocouples, RTDs, and thermistors produce analog signals that require accurate conditioning before digitization. Engineers use low distortion amplifiers to amplify these signals, ensuring that the amplified signal accurately represents the temperature being measured. This accuracy is crucial in applications such as climate control systems, where precise temperature measurements maintain a comfortable and energy-efficient environment.
Pressure Sensors
Pressure sensors measure the pressure of gases or liquids in various applications, including industrial processes, automotive systems, and medical devices. Engineers use low distortion amplifiers to condition the signals from pressure sensors, ensuring that the amplified signal accurately reflects the measured pressure. Accurate pressure measurements maintain safety and efficiency in applications such as hydraulic systems, pneumatic controls, and blood pressure monitors.
Light Sensors
Light sensors, such as photodiodes and phototransistors, measure light intensity in applications like ambient light sensing, optical communication, and imaging systems. Engineers use low distortion amplifiers to amplify signals from light sensors, ensuring that the amplified signal accurately represents the measured light intensity. This accuracy is particularly important in applications like automatic lighting control, where precise light measurements optimize energy usage and maintain a comfortable lighting environment.
Flow Sensors
Flow sensors measure the flow rate of liquids or gases in applications such as water treatment, HVAC systems, and industrial processes. Engineers use low distortion amplifiers to condition signals from flow sensors, ensuring that the amplified signal accurately reflects the measured flow rate. Accurate flow measurements are essential for maintaining process efficiency and ensuring that systems operate within their designed parameters.
Position and Motion Sensors
Position and motion sensors, including accelerometers, gyroscopes, and encoders, measure the position, velocity, and acceleration of objects in applications such as robotics, automotive systems, and consumer electronics. Engineers use low distortion amplifiers to condition signals from these sensors, ensuring that the amplified signal accurately represents the measured position and motion. Accurate position and motion measurements are crucial for applications like navigation, stabilization, and control in systems ranging from drones to industrial robots.
Gas Sensors
Gas sensors detect the presence and concentration of gases in various applications, including environmental monitoring, industrial safety, and medical diagnostics. Engineers use low distortion amplifiers to condition the signals from gas sensors, ensuring that the amplified signal accurately reflects the concentration of the gas being measured. This accuracy is particularly crucial in applications like air quality monitoring, where precise gas measurements are essential for assessing and mitigating the impact of air pollution on public health.
Magnetic Sensors
In applications that involve magnetic sensors, such as Hall effect sensors and magnetoresistive sensors, engineers measure magnetic fields for position sensing, current sensing, and magnetic field mapping. They use low distortion amplifiers to condition the signals from these sensors. Thus they ensure that the amplified signal accurately represents the measured magnetic field. Accurate magnetic field measurements are vital for applications such as motor control, power management, and geomagnetic surveys.
Humidity Sensors
Humidity sensors measure the moisture content in the air for applications such as HVAC systems, weather monitoring, and agricultural control systems. Engineers use low distortion amplifiers to condition the signals from humidity sensors, ensuring that the amplified signal accurately reflects the humidity levels being measured. Accurate humidity measurements help maintain comfort, safety, and efficiency in various environments.
Biosensors
Biosensors detect biological molecules and other analytes in applications like medical diagnostics, environmental monitoring, and food safety. Engineers use low distortion amplifiers to condition the signals from biosensors, ensuring that the amplified signal accurately represents the concentration of the analyte being measured. Precise biosensor measurements play a crucial role in applications such as glucose monitoring, pathogen detection, and biomarker analysis.
Challenges in Designing Low Distortion Amplifiers
Designing low distortion amplifiers for embedded systems presents several challenges that engineers must address to achieve optimal performance.
Thermal Management
Engineers face significant challenges with heat generation in low distortion amplifiers, particularly in class A designs where linearity comes at the cost of efficiency. To prevent thermal drift, which can introduce distortion into the signal, proper thermal management becomes crucial. Engineers use heat sinks, thermal pads, and careful PCB layout design to manage heat dissipation effectively.
Power Consumption
In battery-powered embedded systems, power consumption is a critical concern. Low distortion amplifiers, particularly class A amplifiers, tend to consume more power due to their continuous operation. Designers must strike a balance between achieving low distortion and minimizing power consumption. They should optimize the amplifier’s quiescent current and use power-saving techniques.
PCB Layout Considerations
The layout of the PCB can have a significant impact on the performance of a low distortion amplifier. We must pay careful attention to grounding, signal routing, and the placement of components to minimize noise coupling and crosstalk. Additionally, separating analog and digital grounds, as well as using star grounding techniques, can help reduce noise and distortion.
Cost vs. Performance Trade-offs
High-performance, low distortion amplifiers often come with a higher cost due to the use of precision components and advanced design techniques. Designers must carefully evaluate the cost-performance trade-offs to meet the system’s requirements without exceeding budget constraints.
Conclusion
Low distortion amplifiers are essential components in embedded systems that require high signal fidelity. By minimizing distortion, these amplifiers ensure that signals are accurately processed. This leads to better performance in applications ranging from audio processing to precision measurement and communication systems. Designing low distortion amplifiers involves careful consideration of component selection, circuit topology, feedback mechanisms, and power supply design. We also have to address challenges such as thermal management, power consumption, and PCB layout. As embedded systems continue to evolve and demand higher levels of performance, the role of low distortion amplifiers will only become more critical. This will make them a key focus for designers and engineers alike.
To have a better understanding of IoT Protocols, we recommend using Smowcode to boost your productivity and create great products 10x faster.
Try Smowcode for free and Boost your Productivity by 10x. : https://smowcode.com/
Do go through our other blogs to understand IoT concepts: https://blog.smowcode.com/smart-connectivity-wi-fi-in-the-iot-era/
Link to Modbus Blog: https://blog.smowcode.com/understanding-modbus-in-industrial-iot/