In the realm of embedded systems, where precision and efficiency are crucial, analog multipliers emerge as key components in various signal processing tasks. Specifically, these versatile elements find applications across communications, control systems, instrumentation, and audio processing. Consequently, understanding the operational principles of analog multipliers, selecting the appropriate type for your application, and integrating them effectively into your designs become essential for engineers in this field.
Therefore, this blog will guide you through a comprehensive exploration of analog multipliers. We will delve into their types, applications, performance metrics, and best practices for integration in embedded systems. Whether you are an experienced engineer or a newcomer to the field, this guide will equip you with the knowledge and insights necessary to effectively harness the capabilities of analog multipliers in your projects.
What is an Analog Multiplier?
An analog multiplier is a device that produces an output signal proportional to the product of two input signals. Unlike digital multipliers, which perform multiplication on binary numbers, analog multipliers operate directly on continuous voltage signals. This ability to multiply analog signals makes them indispensable in various applications where signal processing is required.
Mathematically, the operation of an analog multiplier can be expressed as:
Vout=k⋅Vx⋅Vy
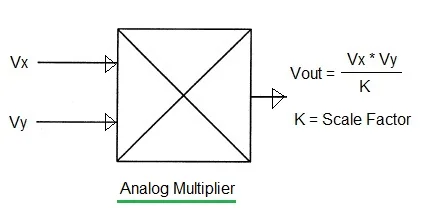
Where:
- VoutV_{out}Vout is the output voltage.
- VxV_xVx and VyV_yVy are the input voltages.
- kkk is the scaling factor, which depends on the design and specifications of the multiplier.
The scaling factor kkk can vary depending on the type of multiplier and its intended application. In some cases, kkk is set to 1, meaning the output is a direct multiplication of the inputs, while in others, kkk might be a fixed value determined by the internal architecture of the multiplier.
The Importance of Analog Multipliers in Embedded Systems
In embedded systems, achieving precise signal manipulation often proves crucial for the desired functionality. For instance, communication systems frequently require modulation or demodulation of signals, which involves multiplying the signals. Likewise, in control systems, proportional-integral-derivative (PID) controllers may need real-time signal multiplication to adjust control signals dynamically.
Consequently, analog multipliers become essential in these scenarios. They allow for the direct multiplication of signals without needing to convert them into digital form. This approach, in turn, simplifies the design process and reduces the latency associated with digital signal processing. Therefore, analog multipliers emerge as a preferred choice for many high-speed and real-time applications.
Types of Analog Multipliers
There are several types of analog multipliers, each with its own strengths and weaknesses. Understanding the differences between these types is crucial for selecting the right multiplier for your application.
Transistor-Based Multipliers
Transistor-based multipliers are among the simplest types of analog multipliers. They leverage the non-linear characteristics of transistors to perform multiplication. The basic principle involves using the transistor’s exponential transfer characteristics. The collector current is an exponential function of the base-emitter voltage.
While transistor-based multipliers are easy to implement, they suffer from limited accuracy and linearity. The non-linear nature of the transistor’s response introduces distortion, making these multipliers suitable only for applications where precision is not a critical requirement.
Operational Amplifier (Op-Amp) Based Multipliers
Operational amplifier-based multipliers leverage the high gain and stability of op-amps to achieve improved accuracy and linearity compared to transistor-based multipliers. By incorporating feedback mechanisms, these multipliers linearize the multiplication process, which results in reduced distortion.
As a result, op-amp-based multipliers are particularly suitable for precision applications, such as instrumentation and control systems. However, despite their advantages, they do exhibit limitations in terms of bandwidth and linearity, especially at higher frequencies. Therefore, while they offer enhanced performance in certain contexts, their suitability may diminish under specific conditions.
Logarithmic Multipliers
Logarithmic multipliers operate on the principle of logarithmic and exponential functions. By taking the logarithm of the input signals, adding them, and then taking the exponent, these multipliers effectively perform multiplication. The mathematical basis for logarithmic multipliers comes from the identity:
log(A⋅B)=log(A)+log(B)\log(A \cdot B) = \log(A) + \log(B)log(A⋅B)=log(A)+log(B)
After computing the logarithm of the input signals, they are summed, and the exponent of the result is taken to obtain the product.
Logarithmic multipliers offer improved accuracy and linearity compared to transistor-based and op-amp-based multipliers. However, they are more complex and expensive, making them suitable for high-precision applications where these characteristics are required.
Gilbert Cell Multipliers
Gilbert cell multipliers are named after the Gilbert cell circuit configuration, a popular topology in RF and communication systems. These multipliers use a differential pair of transistors to achieve multiplication with good linearity and bandwidth.
The Gilbert cell architecture is widely used in RF mixers, modulators, and demodulators, where maintaining signal integrity at high frequencies is essential. These multipliers offer a good balance between accuracy, bandwidth, and complexity, making them ideal for a range of high-frequency applications.
5. Integrated Circuit (IC) Analog Multipliers
Integrated circuit analog multipliers are specialized ICs designed to perform multiplication with high precision and stability. These ICs often incorporate advanced compensation techniques to minimize non-linearity, offset, and temperature drift, making them suitable for demanding applications.
IC analog multipliers are available from various manufacturers, with different specifications to meet the needs of specific applications. They are widely used in instrumentation, communication systems, and control systems, where high accuracy and reliability are required.
Key Performance Metrics
When selecting an analog multiplier for your embedded system, several key performance metrics must be considered. This ensures that the multiplier meets the requirements of your application.
Linearity
Linearity refers to the ability of the multiplier to produce an output that is directly proportional to the product of the inputs over the entire operating range. High linearity is crucial in applications where signal integrity is paramount, such as in RF communication and instrumentation.
Non-linearity in an analog multiplier can introduce distortion into the output signal, which can degrade the performance of the overall system. Linearity is typically expressed as a percentage of the full-scale output, with lower percentages indicating better linearity.
Bandwidth
Bandwidth is the range of frequencies over which the multiplier can accurately perform multiplication. In applications involving high-frequency signals, such as RF communication and audio processing, a wide bandwidth is essential to ensure that the multiplier can handle the full range of input signals without introducing significant attenuation or phase shift.
Bandwidth is often specified as the frequency at which the gain of the multiplier falls to 3 dB below its low-frequency value. For high-frequency applications, multipliers with bandwidths in the hundreds of megahertz or even gigahertz range may be required.
Input and Output Range
The input and output range of an analog multiplier refers to the range of voltages that the multiplier can handle without saturation or clipping. The input range is the range of voltages that can be applied to the inputs without causing the multiplier to enter a non-linear region, while the output range is the range of voltages that the multiplier can produce without distortion.
Selecting a multiplier with an appropriate input and output range is crucial for ensuring accurate signal multiplication in your application. If the input signals exceed the specified range, the multiplier may produce distorted or inaccurate outputs, leading to errors in the overall system.
Power Consumption
Power consumption is a critical factor in embedded systems, especially in battery-powered or energy-efficient designs. Analog multipliers vary in their power consumption depending on their design and application.
For low-power applications, it is essential to select a multiplier that meets the performance requirements while minimizing power consumption. Some multipliers offer power-saving features, such as low-power modes or the ability to dynamically adjust power consumption based on the input signal level.
Noise and Distortion
When designing analog multipliers, noise and distortion are critical factors, especially in high-precision and high-frequency applications. To clarify, noise represents unwanted random fluctuations in the output signal, whereas distortion refers to deviations from the ideal output caused by non-linearities in the multiplier.
Therefore, achieving low levels of both noise and distortion is essential for maintaining signal fidelity. For instance, in audio processing, instrumentation, and RF communication, these low levels ensure that the signal remains accurate and reliable. Consequently, analog multipliers that feature low noise and distortion specifications are preferred for these applications.
Temperature Stability
Temperature stability refers to the ability of the analog multiplier to maintain consistent performance across a range of operating temperatures. The variations in temperature can affect the gain, linearity, and offset of the multiplier, leading to inaccuracies in the output signal.
For applications that operate in harsh or variable environments, selecting a multiplier with good temperature stability is essential to ensure reliable performance. Some IC multipliers include temperature compensation features to minimize the impact of temperature changes on the output signal.
Applications of Analog Multipliers in Embedded Systems
Analog multipliers find extensive use in embedded systems across various applications, including communication, control, instrumentation, and audio processing. Here, we will delve into some of the most common and significant uses of analog multipliers.
Communication Systems
In communication systems, analog multipliers are essential for modulation and demodulation. Modulation involves altering one or more characteristics of a carrier signal, such as amplitude, frequency, or phase, based on a modulating signal. Conversely, demodulation recovers the original modulating signal from the modulated carrier.
Amplitude Modulation (AM)
In amplitude modulation (AM), an analog multiplier plays a pivotal role by adjusting the amplitude of the carrier signal according to the modulating signal. This process multiplies the carrier signal with the modulating signal, producing an amplitude-modulated signal. The modulated signal then travels over a communication channel, like a radio frequency (RF) link.
At the receiver end, another analog multiplier comes into play. This multiplier multiplies the received signal by a local oscillator signal, recovering the original modulating signal. Subsequently, the system processes or decodes this recovered signal for further use.
Frequency Modulation (FM)
In frequency modulation (FM), the frequency of the carrier signal is varied in response to the modulating signal. Although FM typically requires a voltage-controlled oscillator (VCO) rather than a multiplier, analog multipliers can still play a role in certain FM demodulation techniques, such as phase-locked loops (PLLs).
In a PLL-based FM demodulator, an analog multiplier is used as a phase detector to compare the phase of the input signal with that of the VCO. The resulting error signal is used to adjust the VCO frequency, locking it to the frequency of the input signal and recovering the original modulating signal.
Quadrature Amplitude Modulation (QAM)
Quadrature amplitude modulation (QAM) represents a sophisticated modulation technique that integrates both amplitude and phase modulation. Notably, QAM finds extensive application in digital communication systems, including digital television and wireless networks.
In a QAM system, the process begins with two carrier signals, each modulated by distinct components of the modulating signal. These components typically correspond to the in-phase (I) and quadrature (Q) elements. Once modulated, these signals are combined and transmitted. Subsequently, at the receiver end, analog multipliers come into play. They are essential for demodulating the received signal and recovering the I and Q components. Following this, the system decodes these components to retrieve the original data, thus facilitating effective communication.
Signal Processing
Analog multipliers find extensive use in signal processing applications, handling tasks such as frequency mixing, amplitude modulation, and phase detection. These functions are essential in various embedded systems, including audio processing, instrumentation, and control systems.
Phase Detection
In phase detection, engineers measure the phase difference between two signals. Often, analog multipliers serve as phase detectors within phase-locked loops (PLLs), where they compare the phase of the input signal with that of the local oscillator signal.
Within a PLL, the phase detector generates an error signal that is proportional to the phase difference between the input signal and the local oscillator signal. Engineers then filter this error signal and use it to adjust the frequency of the local oscillator, effectively locking it to the frequency of the input signal. PLLs play a critical role in communication systems, frequency synthesis, and clock recovery applications.
Power Measurement
Power measurement is another important application of analog multipliers in embedded systems. By multiplying the voltage and current signals, the multiplier generates a signal proportional to the instantaneous power. This is particularly useful in applications that require real-time monitoring and control of power consumption.
AC Power Measurement
In AC power measurement, engineers use analog multipliers to multiply the instantaneous voltage and current signals, resulting in a signal proportional to the instantaneous power. They then integrate this signal over time to calculate the average power consumption.
Power meters, energy monitoring systems, and smart grid applications commonly rely on AC power measurement. By providing accurate and real-time power measurement, analog multipliers enable efficient energy management and billing.
Power Factor Correction
Power factor correction (PFC) aims to improve the power factor of an AC power system by reducing the phase difference between voltage and current. Analog multipliers play a crucial role in PFC circuits by offering real-time power measurement and control.
In a typical PFC circuit, engineers use the analog multiplier to measure the instantaneous power and generate a control signal that adjusts the phase angle of the current waveform. This adjustment reduces the reactive power in the system, improving the power factor and decreasing energy losses.
Audio Processing
In audio systems, engineers employ analog multipliers for tasks such as amplitude modulation, dynamic range compression, and frequency mixing. These applications require low distortion and high linearity to maintain audio quality and preserve the fidelity of the original sound.
Dynamic Range Compression
Dynamic range compression, a process essential for controlling the dynamic range of an audio signal, works by making the quieter parts louder and the louder parts quieter. To achieve this effect, analog multipliers play a key role in dynamic range compressors. These multipliers adjust the input signal by a gain factor that changes in response to the input signal level.
Furthermore, dynamic range compression finds widespread use in audio recording, broadcasting, and live sound reinforcement. This technique ensures that audio signals stay within a desired range, thereby preventing distortion and enhancing overall sound quality.
Amplitude Modulation
Amplitude modulation is also used in audio processing, particularly in applications such as ring modulation and tremolo effects. Analog multipliers are used to modulate the amplitude of an audio signal, producing effects that vary the volume or create complex harmonic content.
In a ring modulator, for example, an audio signal is multiplied by a low-frequency oscillator (LFO) signal to produce a modulated signal with a unique, metallic sound. Analog multipliers provide the necessary precision and low distortion to achieve high-quality audio modulation.
Frequency Mixing
Frequency mixing represents another critical application of analog multipliers in audio processing. Specifically, in audio synthesis and effects processing, frequency mixing is crucial for combining multiple audio signals or modulating one signal with another.
In this context, analog multipliers facilitate the frequency mixing process by multiplying audio signals. As a result, they create new signals at the sum and difference of the original frequencies. Consequently, this process becomes essential for generating complex sounds. For instance, in frequency modulation (FM) synthesis, a carrier signal undergoes modulation by a modulating signal, leading to rich harmonic content. This harmonic richness adds depth and texture to the sound, enhancing the overall audio experience.
Control Systems
In control systems, engineers use analog multipliers to implement various control strategies, including proportional-integral-derivative (PID) controllers. These controllers rely on real-time signal multiplication to dynamically adjust control signals, thereby enhancing system stability and performance.
Proportional-Integral-Derivative (PID) Controllers
PID controllers are prevalent in industrial control systems, where they work to maintain a desired setpoint by adjusting the control signal based on the error between the setpoint and the measured value. Analog multipliers play a critical role in PID controllers by handling the proportional, integral, and derivative terms.
For the proportional term, engineers multiply the error signal by a constant factor to generate a control signal that is directly proportional to the error. For the integral term, they multiply the accumulated error over time to produce a control signal that addresses long-term deviations. Finally, for the derivative term, engineers multiply the rate of change of the error to create a control signal that anticipates future deviations.
Analog multipliers provide the real-time multiplication needed for PID controllers, ensuring accurate and stable control in diverse applications such as temperature control, motor speed regulation, and process automation.
Adaptive Control
Adaptive control involves adjusting the parameters of the controller in real-time based on changes in system dynamics. In these systems, engineers use analog multipliers to manage real-time parameter adjustment and signal processing.
Specifically, in an adaptive control system, the analog multiplier multiplies the control signal by an adaptive gain factor that adjusts according to the system’s response. This adjustment enables the controller to adapt to changes in the system, thereby improving both performance and stability.
Engineers apply adaptive control in various fields, including robotics, aerospace, and process control, where system dynamics can evolve over time or in response to external disturbances.
Instrumentation
Instrumentation systems often require precise measurement and processing of analog signals, making analog multipliers an essential component in these applications. Analog multipliers are used in instrumentation for tasks such as signal conditioning, measurement, and calibration.
Measurement
Analog multipliers play a crucial role in measurement systems, where they are used for various tasks such as power measurement, frequency measurement, and phase measurement. Since these applications require high accuracy and minimal noise, analog multipliers must meet stringent performance criteria to ensure reliable results.
For example, in a power measurement system, the analog multiplier performs the task of multiplying the voltage and current signals. As a result, this operation generates a signal proportional to the instantaneous power. Following this, the system integrates the signal over time, which allows it to calculate the total energy consumption. Therefore, by ensuring high precision and low noise, analog multipliers significantly contribute to the accuracy and reliability of measurement systems.
Calibration
Calibration is the process of adjusting an instrument or system to ensure accurate and reliable measurements. Analog multipliers are used in calibration systems to generate reference signals, perform signal processing, and adjust measurement parameters.
In a calibration system, the analog multiplier is used to generate reference signals by multiplying a known input signal by a calibration factor. This allows the system to compare the measured signal with the reference signal and adjust the measurement parameters to achieve accurate results.
Best Practices for Using Analog Multipliers in Embedded Systems
Integrating analog multipliers into embedded systems requires careful consideration of various factors to ensure optimal performance. In this section, we will discuss some best practices for using analog multipliers in embedded systems.
Selecting the Right Analog Multiplier
Choosing the right analog multiplier for a specific application is crucial to achieving the desired performance. When selecting an analog multiplier, consider factors such as bandwidth, linearity, input/output impedance, power consumption, and package type.
Bandwidth
Ensure that the analog multiplier’s bandwidth is sufficient for the signals being processed. A wide bandwidth allows the multiplier to handle high-frequency signals without distortion.
Linearity
Choose an analog multiplier with high linearity to minimize distortion and ensure accurate signal multiplication. High linearity is particularly important in applications such as audio processing and communication systems.
Input/Output Impedance
Consider the input and output impedance of the analog multiplier and ensure they are compatible with the surrounding circuitry. Mismatched impedance can lead to signal attenuation or reflection.
Power Consumption
Select an analog multiplier with low power consumption, especially for battery-powered or energy-efficient systems. Low power consumption reduces heat generation and extends battery life.
Package Type
Choose a package type that is suitable for the application’s physical and environmental requirements. Surface-mount packages are commonly used in compact designs, while through-hole packages may be preferred for prototyping or harsh environments.
Designing the Circuit
When designing a circuit that includes an analog multiplier, pay attention to factors such as power supply, grounding, signal routing, and temperature stability.
Power Supply
Provide a stable and noise-free power supply to the analog multiplier. Use decoupling capacitors to filter out any power supply noise and ensure consistent performance.
Grounding
Implement proper grounding techniques to minimize noise and interference. Use a single-point ground or ground plane to reduce ground loops and ensure a clean signal reference.
Signal Routing
Route signals carefully to avoid crosstalk and interference. Keep high-frequency and sensitive signals away from noisy components, and use shielded cables or traces if necessary.
Temperature Stability
Consider the temperature stability of the analog multiplier and the surrounding components. Temperature variations can affect the performance of the analog multiplier, leading to drift or distortion. Use temperature-compensated components or design the circuit to operate within a controlled temperature range.
Testing and Calibration
Thorough testing and calibration are essential to ensure that the analog multiplier performs as expected in the final application.
Functional Testing
Perform functional testing to verify that the analog multiplier operates correctly in the circuit. Test the multiplier’s response to various input signals and ensure that the output signal is accurate and within the expected range.
Performance Testing
Conduct performance testing to evaluate the analog multiplier’s characteristics, such as linearity, bandwidth, noise, and distortion. Compare the results with the manufacturer’s specifications and ensure that the multiplier meets the application’s requirements.
Calibration
Calibrate the analog multiplier and the surrounding circuitry to ensure accurate signal processing. Use precision reference signals and calibration equipment to adjust the circuit parameters and achieve the desired performance.
Conclusion
Analog multipliers are versatile components that play a critical role in various embedded system applications, from signal processing and communication to control systems and instrumentation. Their ability to perform accurate and high-speed signal multiplication makes them indispensable in many modern electronic devices.
Grasping the principles of analog multipliers, along with understanding their various types and applications, is crucial for engineers and designers working in embedded systems. By diligently following best practices in selecting, designing, testing, and calibrating analog multipliers, you can ensure that your embedded system designs achieve optimal performance and reliability.
Whether you’re tackling the design of a communication system, an audio processor, a control system, or an instrumentation device, analog multipliers provide a powerful and versatile solution for a multitude of applications. By fully leveraging the capabilities of analog multipliers, you can significantly enhance the functionality and performance of your embedded systems, enabling them to meet the ever-growing demands of today’s technology-driven world.
To have a better understanding of IoT Protocols, we recommend using Smowcode to boost your productivity and create great products 10x faster.
Try Smowcode for free and Boost your Productivity by 10x. : https://smowcode.com/
Do go through our other blogs to understand IoT concepts: https://blog.smowcode.com/smart-connectivity-wi-fi-in-the-iot-era/
Link to Modbus Blog: https://blog.smowcode.com/understanding-modbus-in-industrial-iot/