In the ever-expanding world of the Internet of Things (IoT), sensor nodes act as the fundamental building blocks. Sensors bridge the digital and physical realms. These nodes capture real-world analog signals, such as temperature, humidity, light, and sound, and convert them into digital data. This data is used for processing and communication. Analog components are integral to this process. They are playing a crucial role in converting, conditioning, and transmitting the data accurately and efficiently.
Much of the focus in IoT development is on the digital aspects, the importance of analog components cannot be overstated. They form the backbone that ensures sensor nodes operate reliably and effectively in diverse environments. Understanding the function of these components is essential for engineers and developers striving to design robust and energy-efficient IoT systems.
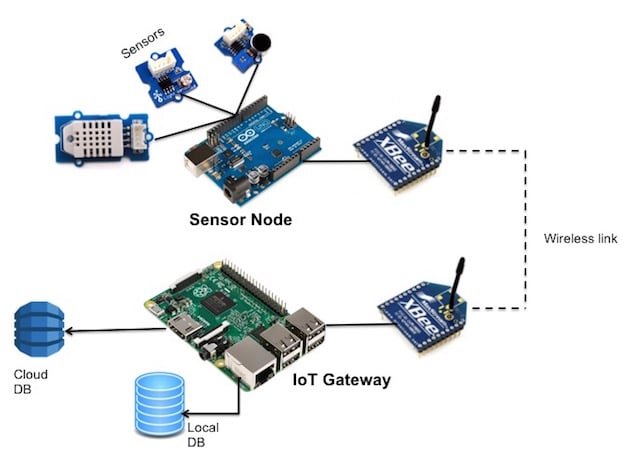
Core Analog Components in IoT Sensor Nodes
At the heart of every IoT sensor node are key analog components that perform several vital functions, from signal conditioning to power management. These components are selected based on the specific requirements of the application. Applications in the electronics industry have very specific requirements such as sensitivity, range, power consumption, and environmental conditions. Each of these components must work in harmony to ensure accurate data collection and energy-efficient operation.
Sensors: The Eyes and Ears of IoT Nodes
Sensors are the primary analog components in any IoT node. They detect physical parameters such as temperature, humidity, pressure, light, and sound, converting them into electrical signals. These signals can be processed by the node’s microcontroller.
Types of Sensors and Their Functionality: Various types of sensors serve different purposes. For instance, thermistors and RTDs are widely used for temperature sensing due to their high sensitivity. Photodiodes and phototransistors measure light intensity, while piezoelectric sensors detect vibrations and sound. Understanding the characteristics of each sensor type — including their range, sensitivity, and response time — is essential for choosing the right sensor for a specific application.
Integration and Calibration: Proper integration and calibration of sensors are crucial to ensure they provide accurate readings. This process involves selecting suitable operational ranges, compensating for environmental factors, and applying corrections to offset any inherent inaccuracies in the sensors. Additionally, choosing sensors with low power consumption is critical in energy-constrained IoT applications.
Operational Amplifiers (Op-Amps): Enhancing Signal Quality
Operational amplifiers, or op-amps, are vital analog components that amplify weak signals from sensors to a level suitable for further processing. They provide functions such as signal amplification, filtering, and conditioning, which are necessary to improve the accuracy and reliability of data.
Signal Amplification: Op-amps amplify the small analog signals generated by sensors, boosting them to levels that can be effectively digitized by Analog-to-Digital Converters (ADCs). Choosing the right gain for the op-amp is essential; too much gain can lead to signal distortion, while too little gain may result in an insufficient signal-to-noise ratio.
Filtering and Noise Reduction: In addition to amplification, op-amps can also be used in analog filters to remove unwanted noise from signals. Low-pass filters eliminate high-frequency noise, while high-pass filters remove low-frequency drift and interference. Designing the right filter circuit ensures that only the desired frequencies are processed, enhancing the overall quality of the data.
Analog-to-Digital Converters (ADCs): Bridging Analog and Digital Worlds
Analog-to-Digital Converters (ADCs) are the linchpins that bridge the analog and digital domains. They convert the amplified analog signals from sensors into digital data that the microcontroller or processor can interpret.
Resolution and Sampling Rate: The resolution of an ADC, typically measured in bits, determines how accurately it can represent the analog signal. Higher-resolution ADCs provide finer granularity, allowing for more precise measurements. The sampling rate, on the other hand, dictates how frequently the ADC samples the signal. A higher sampling rate is necessary for rapidly changing signals, but it also consumes more power.
Power Consumption Considerations: Given the often energy-constrained nature of IoT sensor nodes, selecting ADCs with low power consumption is critical. Delta-Sigma ADCs are known for their high resolution and low noise. This makes them ideal for precision measurements, but they may consume more power than Successive Approximation Register (SAR) ADCs, which offer a good balance between speed, resolution, and power efficiency.
Digital-to-Analog Converters (DACs): Providing Analog Outputs
While ADCs convert analog signals to digital, Digital-to-Analog Converters (DACs) perform the opposite function, converting digital signals back into analog form. DACs are essential in applications where the IoT node needs to control an analog device, such as a motor, actuator, or speaker.
Precision and Control: The precision of a DAC affects how accurately it can reproduce the desired analog signal. High-precision DACs enable fine control over analog outputs, which is particularly important in applications like audio processing or precision instrumentation. Additionally, choosing a DAC with the appropriate output range ensures that it can provide the necessary voltage or current levels for the intended application.
Signal Conditioning Circuits: Preparing Signals for Processing
Signal conditioning circuits are responsible for preparing the raw signals from sensors for accurate conversion and processing. These circuits include components like filters, amplifiers, and level shifters, which adjust the signal to match the input range and characteristics of the ADC.
Filtering and Signal Integrity: To ensure that the signal fed into the ADC is clean and free from noise, low-pass, high-pass, or band-pass filters may be employed. Filters play a crucial role in enhancing signal integrity, especially in environments with significant electrical noise or interference.
Level Shifting: In some cases, the output voltage of a sensor may not be within the acceptable range for the ADC. Level shifters adjust the voltage levels, ensuring compatibility between different components of the circuit. Proper design of these circuits prevents signal clipping or saturation, which could otherwise lead to data inaccuracies.
Key Analog Components and Their Functions
Analog components serve multiple purposes in an IoT sensor node, ranging from sensing and signal processing to power management. Each component contributes to the node’s overall functionality, making them indispensable for reliable operation.
Comparators: Making Real-Time Decisions
Comparators play a fundamental role in IoT sensor nodes for real-time decision-making. They compare two voltages or currents and output a digital signal. This signal shows which input is higher. Many IoT applications need this function for immediate responses to changes.
Applications of Comparators: Comparators are employed in various roles, such as monitoring battery voltage levels, detecting threshold crossings in sensor signals, and generating pulse-width modulation (PWM) signals. In battery-powered IoT devices, for instance, a comparator can shut down non-essential functions when the battery voltage drops below a certain threshold, conserving power.
Challenges and Design Considerations: The primary challenge with comparators is ensuring they operate with minimal power consumption while maintaining high-speed response times. Designers must select comparators with appropriate hysteresis to prevent false triggering due to noise or minor fluctuations in the input signal.
Current Sense Amplifiers: Monitoring Power Consumption
Current sense amplifiers play a vital role in measuring the current flow through various parts of an IoT sensor node. These amplifiers monitor power consumption, provide overcurrent protection, and optimize energy use in battery-powered devices.
Why Current Sense Amplifiers Matter: Efficient power management is critical in IoT applications, especially when devices rely on limited battery life or harvested energy sources. Current sense amplifiers help identify areas where power can be conserved. This enables longer operational lifetimes. Moreover, they can detect anomalies in power usage, indicating potential faults or inefficiencies in the system.
Design Considerations: Choosing a current sense amplifier involves balancing accuracy, bandwidth, and power consumption. Low-offset voltage amplifiers are preferred for high-precision applications, while low-power amplifiers are essential in battery-operated devices. Additionally, designers must carefully select the shunt resistor used in conjunction with the amplifier to ensure minimal impact on the circuit while achieving the desired measurement accuracy.
Phase-Locked Loops (PLLs): Synchronizing Signals
Phase-Locked Loops (PLLs) are critical in IoT sensor nodes that require synchronization of signals, such as those using wireless communication protocols. PLLs are used to generate stable clock signals, demodulate signals, and synchronize frequency, making them indispensable in applications involving data transmission and reception.
PLLs in IoT Communication: In IoT nodes that utilize radio frequency (RF) communication, PLLs help lock the frequency of the local oscillator to the received signal, ensuring accurate data transmission. Moreover, PLLs are used in clock recovery circuits to synchronize data streams in digital communication, which is essential for maintaining data integrity and reducing transmission errors.
Challenges in PLL Design: Designing PLLs involves managing trade-offs between lock time, stability, and power consumption. Fast lock times are crucial for minimizing the time required to establish communication links, while stability is essential for maintaining a reliable connection. Low-power designs are necessary to extend the battery life of IoT nodes.
Bandgap References: Ensuring Voltage Stability
Bandgap references provide a stable reference voltage independent of temperature, supply voltage, and process variations. This stability is essential for the accurate operation of ADCs, DACs, and other analog components that rely on a precise reference voltage.
Importance in IoT Sensor Nodes: In battery-powered IoT devices, maintaining a stable reference voltage is vital for accurate sensor measurements, especially under varying environmental conditions. Bandgap references ensure that the reference voltage remains constant, improving the overall performance and reliability of the sensor node.
Design Challenges: The primary challenge with bandgap references is achieving low power consumption while maintaining accuracy and stability. Designers must carefully select components with low temperature coefficients and minimal drift over time. Additionally, minimizing noise and interference from other parts of the circuit is essential to maintain a clean and stable reference voltage.
Analog Signal Path Optimization
Optimizing the analog signal path is essential for enhancing the performance of IoT sensor nodes. This process involves carefully selecting and designing the analog components that handle sensor signals, ensuring they operate efficiently and accurately.
Matching Impedance for Minimal Signal Loss
Impedance matching is crucial for minimizing signal loss and reflections in the analog signal path. By ensuring the impedance of various components matches the impedance of the transmission line or circuit, designers can prevent signal degradation and maintain data integrity.
Applications of Impedance Matching: In RF communication modules within IoT sensor nodes, impedance matching is vital to maximize signal strength and minimize energy loss. Similarly, when transmitting low-level signals from sensors to amplifiers or ADCs, proper impedance matching reduces noise and distortion, ensuring accurate data conversion.
Challenges and Techniques: The challenge lies in achieving perfect impedance matching across different frequencies and components. Techniques such as using matching networks, transformers, and careful PCB layout design help optimize impedance matching. Additionally, selecting components with compatible impedance ratings simplifies the design process and improves overall performance.
Reducing Crosstalk and Interference
Crosstalk and interference between analog signals can lead to inaccuracies and degraded performance in IoT sensor nodes. Reducing these unwanted interactions is critical for maintaining the quality of the analog signals and ensuring reliable operation.
Strategies for Minimizing Crosstalk: Effective strategies include using proper grounding techniques, maintaining adequate spacing between signal traces, and implementing shielding. Ground planes on PCBs help reduce electromagnetic interference, while twisted pair or coaxial cables are effective for minimizing crosstalk in wired connections. Additionally, using differential signaling can further reduce susceptibility to external noise and interference.
Importance of Signal Isolation: Signal isolation techniques, such as using optocouplers or isolation amplifiers, are crucial when dealing with high-voltage or noisy environments. These techniques prevent high-frequency noise or transients from affecting sensitive analog components, ensuring accurate data collection and processing.
Analog Power Management for IoT Sensor Nodes
Analog components are not only crucial in signal processing but also in managing power effectively in IoT sensor nodes. Optimizing power management involves choosing the right components and designing circuits that maximize energy efficiency and minimize waste.
Power-Factor Correction (PFC) Circuits: Enhancing Efficiency
Power-Factor Correction (PFC) circuits improve the power efficiency of IoT devices by reducing the phase difference between voltage and current. These analog components ensure that the power drawn from the source is used effectively. PFC circuits minimize power losses and improve the overall energy efficiency of the device.
Applications in IoT: PFC circuits are particularly useful in IoT applications that involve motor control, HVAC systems, or any other application where inductive loads are common. They help reduce the total power consumption, contributing to longer battery life or lower operating costs in mains-powered devices.
Challenges in PFC Design: Designing effective PFC circuits requires balancing complexity, cost, and efficiency. Active PFC circuits offer better performance and higher efficiency. They are more complex and expensive compared to passive PFC circuits. Choosing the right type of PFC circuit depends on the specific requirements of the IoT application.
Low-Noise Power Supplies: Ensuring Clean Power
Low-noise power supplies are essential for providing clean, stable power to analog components in IoT sensor nodes. These power supplies minimize ripple and electromagnetic interference (EMI), ensuring that sensitive analog components operate accurately.
Designing Low-Noise Power Supplies: Effective design involves using low-dropout regulators (LDOs) with low output noise, implementing proper filtering techniques, and minimizing noise sources in the circuit layout. Shielding and grounding techniques further help in reducing EMI, ensuring that power supplies remain stable and clean.
Importance in High-Sensitivity Applications: In applications where high sensitivity is required, such as medical devices or precision sensors, the importance of low-noise power supplies cannot be overstated. Any noise in the power supply can directly impact the performance and accuracy of the sensors, leading to erroneous data and potentially dangerous outcomes.
Advanced Analog Techniques for IoT Sensor Nodes
In addition to standard analog components, advanced techniques and strategies are essential for optimizing the performance and efficiency of IoT sensor nodes. These techniques address specific challenges such as power consumption, environmental resilience, and data accuracy.
Dynamic Threshold Adjustment: Adapting to Changing Conditions
Dynamic threshold adjustment involves modifying the threshold levels of comparators and other analog components based on real-time conditions. This technique is particularly useful in applications where environmental conditions vary significantly, such as outdoor monitoring or industrial environments.
Benefits of Dynamic Thresholds: By adjusting the thresholds dynamically, IoT devices can maintain accuracy and reliability under varying conditions. For example, in temperature monitoring applications, dynamic threshold adjustment ensures that the device triggers alarms only under truly critical conditions. This helps in avoiding false positives due to minor fluctuations.
Implementation Strategies: Implementing dynamic threshold adjustment requires careful design of control algorithms and feedback loops. Additionally, the use of programmable components, such as digital potentiometers or microcontrollers, allows for real-time adjustments based on environmental data.
Analog Watchdogs: Monitoring System Health
Analog watchdogs are circuits that monitor the health and status of an IoT sensor node’s analog components. They can detect faults or anomalies, such as excessive noise, signal degradation, or component failures, and trigger appropriate actions to mitigate potential issues.
Applications of Analog Watchdogs: In safety-critical applications, such as medical devices or automotive systems, analog watchdogs provide an additional layer of protection by ensuring that the analog signal path remains within specified parameters. They can shut down or reset the system if any irregularities are detected, preventing malfunctions and ensuring safe operation.
Design Considerations: The design of analog watchdogs involves balancing sensitivity and robustness. They must be sensitive enough to detect small deviations but robust enough to avoid false alarms due to minor fluctuations or noise. Proper calibration and testing are essential to achieve the desired performance.
The Overlooked Importance of Analog Components
Though frequently overshadowed by digital technology, analog components form the backbone of any IoT sensor node. Without the presence of these components, converting real-world signals into digital data would become challenging, if not impossible. Let’s explore how they play their part.
Analog-to-Digital Conversion: More Than Just Conversion
Analog-to-digital converters (ADCs) are the gateway for converting analog signals from the environment into digital signals for processing. However, beyond just digitizing the input, ADCs offer multiple functionalities that make them invaluable in IoT sensor nodes.
Ensuring Signal Fidelity: ADCs maintain signal integrity by accurately capturing and converting the full range of the input analog signal. High-resolution ADCs, for example, can detect subtle changes in sensor outputs, crucial in applications such as environmental monitoring or medical diagnostics, where even minor variations in data are significant.
Noise Reduction and Filtering: Many ADCs include integrated features like anti-aliasing filters, which help reduce noise before conversion. This capability ensures that high-frequency noise does not distort the signal during digitization. For applications like audio processing or vibration analysis, maintaining signal clarity through effective noise reduction is vital.
Low Power Modes for Energy Efficiency: Some ADCs are equipped with low-power modes that can significantly extend battery life, which is especially important for remote IoT devices operating in the field. By shutting down unused parts of the ADC or operating at reduced sampling rates when high resolution is unnecessary, designers can optimize the balance between performance and power consumption.
Operational Amplifiers: Bridging the Gap Between Signals
Operational amplifiers (op-amps) go beyond their traditional role of signal amplification. These components are employed in many applications, from sensor interfacing to signal conditioning and feedback control loops.
Stabilizing Signals: In sensor nodes, op-amps act as buffers, ensuring that signals are stable before they reach the ADC. This function prevents the loading effect that could distort weak sensor signals, ensuring that the microcontroller receives accurate data.
Enabling Precision Measurement: With the ability to handle both very low and high-frequency signals, op-amps enable precision measurement in IoT devices. For example, in a smart grid application, op-amps condition signals from current and voltage sensors to measure power consumption accurately.
Temperature Compensation: Analog circuits often face performance issues due to temperature fluctuations. By using op-amps in differential configurations, IoT designers can create circuits that compensate for temperature-induced variations, maintaining reliable performance across different environments.
Digital Potentiometers: Flexibility in Signal Adjustment
Digital potentiometers, though digital in nature, serve an essential function in analog circuitry by providing adjustable resistance levels. These components are valuable for dynamically tuning and calibrating the analog signal paths within IoT sensor nodes.
Adaptive Gain Control: In applications like audio processing or radio frequency (RF) communication, digital potentiometers dynamically adjust gain levels to accommodate varying signal strengths. This adaptability enhances both signal clarity and device reliability.
Automatic Calibration: For IoT devices that must adapt to changing environments, digital potentiometers facilitate automatic calibration of analog circuits. This feature is particularly useful in applications like environmental monitoring. Here, sensor calibration must be adjusted based on temperature, humidity, or other external factors.
Implementing Feedback Control: Feedback control loops in analog circuits often require precise tuning to ensure stable operation. Digital potentiometers offer an efficient means to fine-tune these loops. This will be allowing for precise control over parameters such as sensor biasing, gain, or offset levels.
Enhancing Power Management Through Analog Design
Power management is a critical aspect of IoT sensor nodes, especially those operating on battery or harvested energy sources. Analog components offer several strategies to enhance power efficiency, prolonging the device’s operational lifetime.
Linear Regulators: Simple Solutions for Low-Noise Power
Linear regulators, or Low Dropout Regulators (LDOs), provide a stable voltage supply to sensitive analog components.They minimise noise and ripple that could affect sensor accuracy.
Low Noise Characteristics: Unlike switching regulators, LDOs generate minimal electromagnetic interference (EMI). EMI is crucial for IoT nodes that rely on low-level signal acquisition. For instance, in medical applications like wearable health monitors, using LDOs ensures that the analog front-end remains free from digital switching noise. This enhances the reliability of vital signal measurements.
Reduced Complexity and Size: The simplicity of linear regulators reduces the overall circuit complexity, making them suitable for compact IoT designs where space is at a premium. Their use minimizes the number of external components, reducing the footprint of the power supply circuit.
Thermal Management: Since LDOs inherently dissipate excess energy as heat, their design must carefully consider thermal management, especially in high-power IoT applications. While this could be a limitation, in applications requiring low current and low noise, LDOs are unmatched in performance.
Charge Pumps: Efficient Voltage Conversion
Charge pumps are DC-DC converters that provide efficient voltage conversion without the need for inductors. These components offer several advantages in the power management of IoT sensor nodes.
Compact Form Factor: The lack of inductors makes charge pumps ideal for compact IoT devices where board space is limited. Charge pumps can step up, step down, or invert voltages with minimal external components, saving space and simplifying the design.
Low Quiescent Current: Charge pumps generally exhibit low quiescent current, which is advantageous in battery-operated IoT devices. By minimizing power consumption when the device is idle, charge pumps help extend battery life, making them suitable for long-term monitoring applications.
Multi-Output Capability: Some charge pumps offer multiple outputs, allowing designers to generate different voltage levels from a single input source. This flexibility is particularly useful in IoT applications where multiple components, each requiring a different voltage, must be powered from a single battery.
Advanced Analog Signal Conditioning Techniques
Signal conditioning plays a vital role in preparing raw sensor outputs for accurate analog-to-digital conversion. Advanced analog techniques help enhance signal fidelity, reduce noise, and compensate for environmental variations.
Active Filtering: Improving Signal-to-Noise Ratio (SNR)
Active filters, which use op-amps in their design, offer several advantages over passive filters in IoT sensor nodes.
Adaptive Filtering: By using programmable analog components, designers can create filters that adapt to changing signal conditions. For instance, in an IoT air quality monitor, active filters can dynamically adjust their cutoff frequency. This is done to compensate for varying environmental noise levels.
Minimizing Power Consumption: Active filters can operate at lower voltages compared to passive filters, reducing overall power consumption. This characteristic is particularly beneficial in battery-powered IoT devices that require prolonged operational lifetimes.
Enhancing Selectivity: The high selectivity of active filters allows them to isolate specific frequency bands with greater precision, ensuring that only the desired signal components reach the ADC. This is particularly useful in applications such as RF communication, where distinguishing between closely spaced channels is essential.
Analog Multiplexing: Efficient Sensor Integration
Analog multiplexers enable multiple sensors to share a single ADC, significantly reducing the cost and complexity of IoT sensor nodes.
Optimizing Resource Utilization: By allowing multiple sensors to connect to a single ADC, analog multiplexers optimize resource utilization, reducing both the number of components and power consumption. This is particularly beneficial in applications where space and power are limited, such as wearable devices or implantable medical sensors.
Dynamic Signal Selection: Analog multiplexers can dynamically select signals based on predefined criteria, enabling more intelligent data acquisition strategies. For example, in a smart agricultural system, the multiplexer could prioritize moisture sensor readings during irrigation cycles and temperature sensor readings at other times.
Minimizing Signal Degradation: Careful design of the analog multiplexer circuit, including considerations for switching speed, impedance matching, and signal isolation, ensures minimal signal degradation during switching. This guarantees that sensor data remains accurate and reliable even when multiple channels are being monitored.
Emphasizing Environmental Resilience in IoT Analog Design
Environmental factors such as temperature, humidity, and electromagnetic interference can significantly impact the performance of analog components in IoT sensor nodes. To maintain reliability and accuracy, analog designs must account for these external conditions.
Temperature Compensation Techniques: Stability Across Environments
Analog components are often sensitive to temperature variations, which can affect their performance. To mitigate these effects, designers employ temperature compensation techniques that ensure stable operation across a wide range of environments.
Using Temperature Coefficients: Selecting components with low-temperature coefficients ensures that their performance remains consistent across varying temperatures. In precision applications like industrial automation or medical devices, this consistency is crucial for maintaining accuracy and reliability.
Thermistor-Based Compensation: By incorporating thermistors into analog circuits, designers can dynamically adjust parameters such as gain, offset, or bias voltage in response to temperature changes. This technique is particularly effective in battery-powered IoT devices that operate outdoors, where temperatures can vary significantly.
Compensation Through Calibration: Regular calibration using reference signals or external sensors can also help compensate for temperature-induced drift. By periodically recalibrating, IoT devices can maintain their accuracy over time, even as environmental conditions fluctuate.
Shielding and Grounding: Protecting Against Interference
Electromagnetic interference (EMI) can degrade the performance of analog circuits in IoT sensor nodes, leading to signal loss or corruption. Proper shielding and grounding techniques are essential for protecting against these unwanted effects.
Implementing Shielded Enclosures: By enclosing sensitive analog components in shielded housings, designers can prevent EMI from external sources, such as RF transmitters or power lines, from affecting the signal integrity. This practice is especially important in urban IoT deployments, where multiple wireless devices may operate in close proximity.
Optimizing Grounding Schemes: Effective grounding schemes, including star or ground plane configurations, minimize ground loops and reduce noise in analog circuits. This technique ensures that the sensor node operates reliably, even in electrically noisy environments.
Power Management in IoT Sensor Nodes
Power management is another critical area where analog components shine. IoT sensor nodes typically rely on battery power or harvested energy, necessitating circuits that minimize power consumption while maximizing operational life.
Voltage Regulators: Ensuring Stable Power Supply
Voltage regulators maintain a stable output voltage despite variations in the input supply or load conditions. They are essential in IoT sensor nodes to ensure that sensitive analog and digital components receive a consistent voltage level, preventing malfunction or damage due to power fluctuations.
Low Dropout Regulators (LDOs): LDOs are commonly used in low-power IoT applications due to their simplicity and efficiency in providing a stable voltage output with minimal drop from the input voltage. However, choosing an LDO with low quiescent current is vital to avoid unnecessary power drain in battery-operated devices.
Switching Regulators: Switching regulators, including buck and boost converters, are ideal for applications requiring high efficiency across a wide range of input voltages. They can step up or step down the input voltage as needed, providing flexibility in energy harvesting applications where the input voltage may fluctuate.
Energy Harvesting Circuits: Maximizing Available Power
Energy harvesting circuits capture ambient energy from sources like solar, thermal, vibration, or RF signals and convert it into electrical power to charge batteries or capacitors. These circuits are essential for enabling long-term operation of IoT nodes in remote or hard-to-reach locations.
MPPT Controllers: Maximum Power Point Tracking (MPPT) controllers optimize the power extracted from energy sources, such as solar panels or thermoelectric generators. By continuously adjusting the operating point, MPPT controllers ensure maximum efficiency in energy conversion.
Power Storage Management: In addition to energy harvesting, power management circuits control the charging and discharging of storage elements, such as supercapacitors or rechargeable batteries. These circuits must prevent overcharging or deep discharging.This can reduce the lifespan of the storage elements and degrade performance over time.
Design Challenges and Considerations
Designing IoT sensor nodes with efficient analog components involves navigating several challenges, from power consumption to environmental factors. Addressing these challenges requires a holistic approach that balances performance, power, size, and cost.
Power Efficiency vs. Performance
One of the primary trade-offs in designing IoT sensor nodes is achieving power efficiency while maintaining acceptable performance. Since many IoT devices are battery-powered, every component must be carefully chosen. By the correct choice, we can minimize power consumption without sacrificing functionality.
Component Selection and Trade-offs: Choosing low-power analog components, such as ultra-low-power op-amps and ADCs, helps extend battery life. However, designers must also consider factors like noise, accuracy, and speed. For instance, a lower-power ADC may have a lower sampling rate or higher noise floor, which could impact the quality of the measurements.
Environmental Considerations
IoT sensor nodes often operate in harsh environments. Here, factors such as temperature, humidity, and mechanical stress can affect their performance. Analog components must be robust and designed to withstand these conditions.
Temperature Compensation and Calibration: Temperature variations can cause drift in sensor readings and circuit performance. To counteract this, incorporate temperature compensation techniques, we can use thermistors or temperature-stable components. We can also calibrate the sensors periodically to maintain accuracy.
Durability and Reliability: Selecting components with a wide operating temperature range and resistance to humidity and corrosion is crucial for outdoor or industrial applications. Robust packaging and conformal coatings can also help protect sensitive analog components from environmental damage.
Size and Form Factor Constraints
With the trend towards miniaturization in IoT devices, size and form factor are significant considerations in analog circuit design. The challenge lies in integrating all necessary analog components into a compact, low-profile package.
Integration of Analog Components: Using integrated circuits (ICs) that combine multiple functions. Some useful ICs are op-amps, ADCs, and voltage regulators — can reduce the size and complexity of the circuit. Additionally, employing surface-mount technology (SMT) components minimizes the overall footprint and weight, making the design suitable for space-constrained applications.
Conclusion
Analog components play a fundamental role in the effective operation of IoT sensor nodes. This enables these devices to interact seamlessly with the physical world. From sensors and amplifiers to converters and power management circuits, these components ensure accurate signal processing, efficient power usage, and reliable operation in various environments.
By understanding the roles and challenges associated with these analog components, designers can create IoT sensor nodes that are not only efficient and powerful but also adaptable to the diverse needs of today’s interconnected world. As IoT technology continues to advance, the importance of mastering analog design principles will only grow. This will be driving innovation and enabling new applications across industries.
To have a better understanding of IoT Protocols, we recommend using Smowcode to boost your productivity and create great products 10x faster.
Try Smowcode for free and Boost your Productivity by 10x. : https://smowcode.com/
Do go through our other blogs to understand IoT concepts: https://blog.smowcode.com/smart-connectivity-wi-fi-in-the-iot-era/
Link to Modbus Blog: https://blog.smowcode.com/understanding-modbus-in-industrial-iot/