In the realm of wireless communication technologies, Zigbee stands out for its simplicity, low power consumption, and robustness. Designed specifically for low-rate wireless personal area networks (LR-WPANs), Zigbee has become a cornerstone in the Internet of Things (IoT) ecosystem. It enables seamless communication between a wide variety of smart devices, making it an ideal choice for applications ranging from home automation to industrial control systems. This blog delves into the intricacies of Zigbee wireless communication, exploring its history, functionality, architecture, and applications, as well as its significance in modern IoT systems.
The Genesis of Zigbee
Zigbee was conceived in the late 1990s as a solution for low-power, low-data-rate wireless communication. The Zigbee Alliance, an association of companies that promotes the development of Zigbee standards, was officially formed in 2002. The first Zigbee specification was released in 2004, and since then, it has undergone several enhancements to address the evolving needs of the market.
Zigbee was designed to operate in the unlicensed ISM (Industrial, Scientific, and Medical) radio bands, including 2.4 GHz, 900 MHz, and 868 MHz. Its primary goal was to provide a reliable and cost-effective solution for wireless communication in applications where low power consumption and long battery life are crucial.
General Characteristics of Zigbee Standard
- Low Power Consumption
- Low Data Rate (20- 250 kbps)
- Short-Range (75-100 meters)
- Network Join Time (~ 30 msec)
- Support Small and Large Networks (up to 65000 devices (Theory); 240 devices (Practically))
- Low Cost of Products and Cheap Implementation (Open-Source Protocol)
- Extremely low-duty cycle.
- 3 frequency bands with 27 channels.
Operating Frequency Bands
(Only one channel will be selected for use in a network)
- Channel 0: 868 MHz (Europe)
- Channel 1-10: 915 MHz (the US and Australia)
- Channel 11-26: 2.4 GHz (Across the World)
The Fundamentals of Zigbee Communication
Network Topologies
Star Topology
In a star topology, all devices, also known as end devices, communicate directly with a central device called the coordinator. The coordinator acts as the hub of the network, managing communication and data flow between the devices.
Advantages
- Simplicity: The star topology is straightforward to implement and manage. With all devices connecting to a single central point, network setup and maintenance are relatively simple.
- Low Latency: Because all communication is routed through the central coordinator, the path between any two devices is direct. This minimizes latency, making it suitable for applications that require quick response times.
- Centralized Control: The coordinator can efficiently manage network resources and security, ensuring that data integrity and network performance are maintained.
Disadvantages
- Limited Range: The overall network range is limited to the transmission range of the coordinator. Devices that are too far from the coordinator cannot participate in the network.
- Scalability Constraints: The number of devices that can be effectively managed by a single coordinator is limited, restricting the network’s scalability.
- Single Point of Failure: If the coordinator fails, the entire network becomes inoperative, posing a significant risk to network reliability.
Applications
Star topology is best suited for small networks where simplicity and low latency are critical. Examples include smart home systems, small office automation, and personal area networks.
Tree Topology
In a tree topology, devices are organized in a hierarchical structure. The network begins with a central coordinator, and devices are connected in parent-child relationships, creating branches that extend from the root to the leaves.
Advantages
- Extended Range: The hierarchical structure allows the network to cover a larger area. Devices can communicate with their parent and child devices, effectively extending the range beyond the immediate vicinity of the coordinator.
- Improved Scalability: Tree topology supports more devices than a star topology because it can grow in multiple directions. Each parent device can manage several child devices, creating a scalable network.
- Structured Organization: The hierarchical arrangement helps in organized data routing and resource allocation. Data can be aggregated at intermediate nodes before reaching the coordinator, optimizing network efficiency.
Disadvantages
- Complexity: Setting up and managing a tree topology is more complex than a star topology. Proper planning is required to ensure efficient data routing and avoid bottlenecks.
- Latency: Communication between distant devices may need to pass through several intermediate nodes, increasing latency.
- Reliability Issues: If a parent node fails, all its child devices lose their connection to the network until a new route is established, potentially causing disruptions.
Applications
Tree topology is ideal for applications that require extended coverage and scalability, such as building automation, industrial monitoring, and utility management.
Mesh Topology
Mesh topology is the most robust and flexible of the three. In a mesh network, each device can communicate with multiple other devices, creating multiple pathways for data to travel. This redundancy ensures that the network can self-heal and reroute data in case of node failures.
Advantages
- High Reliability: The multiple pathways in a mesh network provide redundancy. If one path fails, data can be rerouted through alternative paths, ensuring continuous communication.
- Scalability: Mesh topology can support a large number of devices. The network can grow dynamically as more devices are added, with each new device extending the network’s coverage.
- Range Extension: Devices can relay data for other devices, extending the network’s range far beyond the limitations of individual devices’ transmission ranges.
- Self-Healing: Mesh networks are inherently resilient. They can automatically reconfigure themselves when nodes are added or removed, maintaining network integrity without manual intervention.
Disadvantages
- Complexity: Mesh networks are complex to design and manage. Routing algorithms and network maintenance require sophisticated protocols and management tools.
- Increased Latency: While mesh networks are reliable, data may need to hop through several devices before reaching its destination, potentially increasing latency.
- Higher Power Consumption: Devices in a mesh network may need to stay active for longer periods to relay data, leading to higher power consumption compared to star and tree topologies.
Applications
Mesh topology is well-suited for large-scale, mission-critical applications where reliability and coverage are paramount. Examples include smart grids, large-scale industrial automation, military communications, and city-wide sensor networks.
Device Types
Zigbee is a widely adopted wireless communication protocol designed for low-power, low-data-rate applications, particularly in the domains of home automation, industrial control, and sensor networks. The architecture of a Zigbee network consists of three primary types of devices: coordinators, routers, and end devices. Each device plays a distinct role in the network, contributing to its overall functionality, efficiency, and robustness. Here, we delve into the specific roles and characteristics of these devices, highlighting how they interact to create a cohesive and efficient Zigbee network.
Coordinator
The coordinator is the cornerstone of a Zigbee network, serving as the central control point and the most capable device in the network. There is only one coordinator in each Zigbee network, and its primary responsibilities include initializing, managing, and maintaining the network.
Responsibilities and Functions
- Network Initialization: The coordinator is responsible for creating and initializing the Zigbee network. This includes setting up the network parameters, such as the channel and the unique network identifier (PAN ID).
- Address Assignment: One of the critical tasks of the coordinator is to assign unique addresses to all devices joining the network. This ensures that each device can be distinctly identified and addressed within the network.
- Security Management: The coordinator manages the security settings of the network, including encryption keys and authentication mechanisms, ensuring secure communication between devices.
- Routing and Data Management: While the coordinator itself can route data, its primary role is to manage the overall data flow within the network, ensuring efficient and reliable communication.
Characteristics
- High Processing Power: The coordinator typically has more processing power and memory compared to other devices in the network, enabling it to handle complex tasks and manage large amounts of data.
- Permanent Power Supply: Unlike end devices, the coordinator is usually connected to a permanent power supply, allowing it to operate continuously without concerns about battery life.
Applications
Coordinators are essential in applications requiring centralized control and monitoring, such as smart home hubs, industrial control systems, and large-scale sensor networks.
Router
Routers play a vital role in extending the range and enhancing the robustness of a Zigbee network. They relay data between devices, including the coordinator, other routers, and end devices, thus ensuring that communication can occur over greater distances and around obstacles.
Responsibilities and Functions
- Data Relay: The primary function of a router is to relay data packets from one device to another. This capability extends the communication range of the network and ensures that devices located far from the coordinator can still communicate effectively.
- Network Extension: By adding routers to a network, the overall coverage area can be significantly increased, enabling communication in larger or more complex environments.
- Route Discovery and Maintenance: Routers participate in the dynamic discovery and maintenance of routes within the network, ensuring that data packets can find the most efficient path to their destination.
Characteristics
- Intermediate Processing Power: Routers typically have moderate processing power and memory, sufficient to handle routing tasks and relay data without requiring the high capabilities of a coordinator.
- Permanent or Semi-Permanent Power Supply: Routers often have access to a more reliable power source than end devices, though they may still need to manage their power consumption carefully.
Applications
Routers are crucial in applications where extended coverage and reliable communication are necessary, such as in industrial automation, agricultural monitoring, and large-scale building management systems.
End Device
End devices are the most numerous in a Zigbee network, designed to be low-power devices that interact with the network through either the coordinator or a router. They are typically used for sensing, control, and user interaction.
Responsibilities and Functions
- Data Generation and Consumption: End devices primarily generate data (e.g., sensor readings) or consume data (e.g., actuation commands) without relaying it to other devices. This helps in conserving their battery life.
- Low Power Operation: End devices are optimized for low power consumption, often operating on battery power and employing sleep modes to extend battery life.
Characteristics
- Minimal Processing Power: End devices have the least processing power and memory in the network, sufficient for their specific tasks but not for data routing.
- Battery-Powered: Most end devices rely on battery power, necessitating efficient energy management to maximize their operational life.
Applications
End devices are ubiquitous in applications such as home automation (e.g., smart thermostats, light switches), environmental monitoring (e.g., temperature and humidity sensors), and personal health devices (e.g., wearable fitness trackers).
Integration and Interaction
The successful operation of a Zigbee network relies on the seamless integration and interaction of coordinators, routers, and end devices. Each device type plays a specific role that contributes to the network’s overall functionality and efficiency. The coordinator establishes and manages the network, routers extend its range and robustness, and end devices perform the sensing and actuation tasks necessary for the application.
Architecture of Zigbee Wireless Communication
Zigbee architecture is built on the IEEE 802.15.4 standard, which defines the physical and media access control (MAC) layers. Zigbee adds network, security, and application layers on top of these to create a complete protocol stack suitable for wireless communication. The key components of Zigbee architecture include:
Physical Layer (PHY)
Frequency Bands: Zigbee operates in the 2.4 GHz ISM band globally, with additional frequencies (868 MHz in Europe and 915 MHz in the U.S.) providing flexibility in various regions.
Modulation and Data Rates: The physical layer uses Direct Sequence Spread Spectrum (DSSS) and Offset Quadrature Phase-Shift Keying (O-QPSK) modulation, supporting data rates up to 250 kbps.
Media Access Control (MAC) Layer
Channel Access: The MAC layer employs Carrier Sense Multiple Access with Collision Avoidance (CSMA/CA) to manage access to the communication channel and minimize collisions.
Frame Structure: It defines the frame structure for data, acknowledgment, beacon, and command frames, ensuring reliable communication.
Network Layer
Routing: The network layer handles routing, addressing, and network formation. It supports star, tree, and mesh topologies, providing flexibility in network design.
Network Discovery and Formation: Devices can discover and join existing networks or form new ones, with the coordinator playing a central role in network management.
Application Layer
Application Support Sub-layer (APS): This sub-layer provides data services, binding tables, and group management, enabling communication between devices at the application level.
Zigbee Device Objects (ZDO): ZDOs define device roles, capabilities, and services, facilitating interoperability between different Zigbee devices.
Application Framework: The application framework supports multiple application profiles, allowing for a wide range of use cases and ensuring compatibility between devices from different manufacturers.
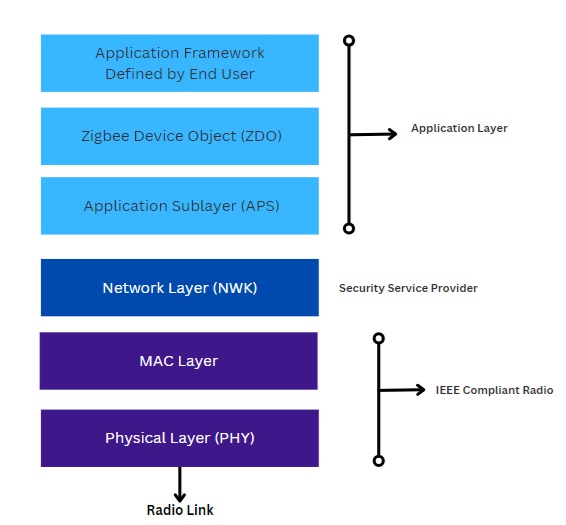
Security and Encryption
Security is paramount in Zigbee communication, especially considering its widespread use in applications ranging from home automation to industrial control systems. Zigbee incorporates several robust security mechanisms designed to ensure data integrity, confidentiality, and authenticity. These features protect against unauthorized access and ensure that communications within the network remain secure and trustworthy.
AES-128 Encryption
At the core of Zigbee’s security framework is the Advanced Encryption Standard (AES) with a 128-bit key. AES-128 is a highly secure encryption algorithm that has been widely adopted across various industries for protecting sensitive data. In Zigbee, AES-128 encryption ensures that data transmitted over the network is encrypted, making it accessible only to authorized devices.
Benefits of AES-128 Encryption
- Data Confidentiality: By encrypting the data, Zigbee ensures that any intercepted communications cannot be read by unauthorized entities.
- Data Integrity: AES-128 helps in maintaining the integrity of the data, ensuring that it has not been altered or tampered with during transmission.
- Data Authenticity: Encryption also plays a role in verifying that the data originates from a legitimate source.
Network and Link Keys
Zigbee employs a dual-key mechanism to manage encryption and ensure secure communication across the network. These keys are essential for maintaining both broad network security and specific device-to-device communication.
Network Keys
- Shared Security: Network keys are common to all devices within a Zigbee network. They are used to encrypt broadcast communications, ensuring that only devices within the network can decipher the transmitted information.
- Ease of Management: Using a single network key simplifies key management and distribution, particularly in large networks.
Link Keys
- Pairwise Security: Link keys are unique to each pair of devices communicating directly with each other. This ensures that even if the network key is compromised, direct communications between devices remain secure.
- Enhanced Privacy: By using unique link keys, Zigbee ensures that sensitive data exchanged between two devices is protected from other devices within the same network.
Key Management
Effective key management is critical for maintaining the security of Zigbee networks. Zigbee includes sophisticated mechanisms for the secure distribution, storage, and updating of keys.
Key Distribution
- Secure Joining: When a new device joins the network, Zigbee ensures that keys are securely distributed to the device, preventing unauthorized access during the joining process.
- Key Transport: Zigbee protocols facilitate the secure transport of keys across the network, ensuring that keys cannot be intercepted or compromised.
Key Updating
- Dynamic Updates: Zigbee supports the regular updating and rotating of keys to minimize the risk of long-term key exposure.
- Re-keying Mechanisms: Devices can initiate re-keying processes to update their encryption keys, ensuring continued security even if a key is suspected to be compromised.
Applications of Zigbee
Home Automation
Zigbee is widely used in home automation systems for controlling lights, thermostats, security systems, and other smart home devices. Its low power consumption and robust communication make it ideal for battery-powered devices that need to operate reliably over long periods.
Industrial Automation
In industrial settings, Zigbee is used for monitoring and controlling machinery, processes, and systems. Its mesh topology and robust security features make it suitable for environments where reliability and data integrity are paramount.
Smart Metering
Zigbee is a popular choice for smart metering applications, enabling utilities to remotely monitor and manage energy consumption. Zigbee-enabled smart meters can communicate with home energy management systems and provide real-time data to utilities, helping to optimize energy usage and reduce costs.
Healthcare
In healthcare, Zigbee is used for wireless medical devices that monitor patients’ vital signs and transmit data to healthcare providers. Its low power consumption and reliable communication are critical for devices that need to operate continuously without frequent battery replacements.
Agriculture
Zigbee is used in precision agriculture for monitoring soil moisture, temperature, and other environmental parameters. This data helps farmers optimize irrigation, reduce water usage, and improve crop yields.
Building Automation
Zigbee is employed in building automation systems for controlling HVAC, lighting, and security systems. Its mesh topology allows for easy expansion and reconfiguration, making it suitable for large and complex buildings.
Advantages of Zigbee
Low Power Consumption
One of the primary advantages of Zigbee is its low power consumption. Zigbee devices are designed to operate for years on a single battery, making them ideal for applications where long battery life is essential.
Scalability
Zigbee networks can scale to support hundreds of devices, making them suitable for both small and large installations. The mesh topology ensures that the network can be easily expanded without significant changes to the infrastructure.
Reliability
The mesh topology of Zigbee networks enhances their reliability by providing multiple pathways for data to travel. If one path fails, data can be rerouted through another path, ensuring continuous communication.
Cost-Effectiveness
Zigbee is a cost-effective solution for wireless communication. Its simplicity and low power consumption reduce the overall cost of devices and installation, making it an attractive option for a wide range of applications.
Interoperability
The Zigbee Alliance has developed a set of standards and certification programs to ensure interoperability between Zigbee devices from different manufacturers. This interoperability allows for the integration of various devices into a single network, providing a seamless user experience.
Challenges and Considerations
Interference
Zigbee operates in the 2.4 GHz Industrial, Scientific, and Medical (ISM) band, which is a popular frequency range utilized by various wireless technologies, including Wi-Fi, Bluetooth, and cordless phones. While this band offers global availability and is unlicensed, it is also highly congested, leading to potential interference issues that can impact Zigbee’s performance.
In environments with many wireless devices operating in the 2.4 GHz band, such as smart homes or industrial settings, interference can cause packet loss, increased latency, and reduced overall network performance. Zigbee employs Direct Sequence Spread Spectrum (DSSS) to mitigate interference, but it cannot entirely eliminate the problem, especially in very dense wireless environments.
One way to minimize interference is through careful planning of the network layout and channel allocation. Zigbee networks can operate on different channels within the 2.4 GHz band, and selecting a channel with minimal overlap with other devices can help reduce interference. Additionally, using a dual-band router that operates on both 2.4 GHz and 5 GHz frequencies for Wi-Fi can alleviate congestion on the 2.4 GHz band.
Range Limitations
Zigbee’s individual devices typically have a shorter communication range compared to other wireless technologies like Wi-Fi. A typical Zigbee device might have a range of 10 to 100 meters indoors, depending on the environment and obstacles. This limitation can pose challenges in large buildings or industrial sites where devices need to communicate over long distances.
However, Zigbee’s mesh network topology can mitigate this limitation. In a mesh network, each Zigbee device can act as a router, forwarding data to other devices. This allows the network to cover a much larger area than would be possible with a single device. The range can be effectively extended by strategically placing additional routers throughout the network.
Despite this advantage, adding more routers can increase the complexity and cost of the network. Moreover, each additional hop in the network can introduce slight delays, which might be a consideration for time-sensitive applications. Therefore, proper network planning is crucial to balance range extension and performance.
Data Rate
Zigbee’s maximum data rate of 250 kbps is designed to be sufficient for many low-bandwidth IoT applications such as sensor networks, home automation, and industrial monitoring. This data rate supports typical IoT communication needs, including sending small packets of data like temperature readings, light levels, or device status updates.
However, for applications that require higher data throughput, such as video streaming or large data transfers, Zigbee’s data rate might be inadequate. This limitation necessitates the use of other wireless technologies like Wi-Fi, which offers much higher data rates but at the cost of higher power consumption.
For applications with mixed data requirements, a hybrid approach might be necessary. For example, a smart home system could use Zigbee for low-power, low-bandwidth devices like sensors and Wi-Fi for high-bandwidth needs like security cameras. This approach leverages the strengths of each technology while addressing their limitations.
Complexity
Implementing and managing a Zigbee network, especially in large installations with numerous devices, can be complex. Zigbee networks require careful planning and configuration to ensure optimal performance and reliability. Factors such as network topology, device placement, channel selection, and interference management all play crucial roles in network setup.
Network planning must consider the placement of the coordinator, routers, and end devices to ensure effective communication and coverage. The coordinator should be placed centrally to manage the network efficiently, while routers should be positioned to create a robust mesh network that can handle data traffic and extend the network’s range.
Configuring a Zigbee network involves setting up each device, assigning unique addresses, and ensuring proper routing paths are established. This process can be time-consuming and requires technical expertise, especially in environments with many devices. Moreover, maintaining the network involves monitoring performance, managing interference, and potentially reconfiguring devices as network demands change.
Security Considerations
In addition to the operational challenges, security is a critical aspect of managing a Zigbee network. Zigbee employs AES-128 encryption to secure communications, but network administrators must ensure that security features are correctly implemented and maintained. This includes managing encryption keys, ensuring firmware updates to patch vulnerabilities, and monitoring for unauthorized access.
Scalability
Scalability is another important consideration for Zigbee networks. While the mesh topology inherently supports scalability, adding a large number of devices can introduce network management challenges. As the network grows, ensuring efficient communication and maintaining performance becomes more complex. Advanced network management tools and strategies, such as hierarchical routing and efficient addressing schemes, can help manage large-scale Zigbee deployments.
Conclusion
Zigbee wireless communication offers a robust, scalable, and cost-effective solution for a wide range of IoT applications. Its low power consumption, mesh topology, and robust security features make it an ideal choice for home automation, industrial control, smart metering, healthcare, agriculture, and building automation. Despite challenges such as interference and range limitations, Zigbee’s advantages far outweigh its drawbacks, making it a cornerstone technology in the evolving landscape of wireless communication. As IoT continues to grow, Zigbee’s role in enabling seamless, reliable, and efficient communication between smart devices will remain vital.
The adaptability of Zigbee makes it an essential tool in the ever-growing IoT landscape. In home automation, it seamlessly integrates various smart devices, enabling efficient control and monitoring of everything from lighting to security systems. In industrial settings, it supports complex automation processes, improving efficiency and reducing operational costs. In agriculture, Zigbee networks help monitor soil moisture, weather conditions, and crop health, contributing to more sustainable farming practices.
As IoT continues to expand, the role of Zigbee in enabling reliable and efficient communication between smart devices will only become more critical. Its combination of low power consumption, robust security, and flexible network architecture ensures that it remains a cornerstone technology in the world of wireless communication, driving innovation and connectivity across multiple sectors.
Try Smowcode for free and Boost your Productivity by 10x. : https://smowcode.com/
Do go through our other blogs to understand IoT concepts: https://blog.smowcode.com/smart-connectivity-wi-fi-in-the-iot-era/
USB: https://blog.smowcode.com/usb-protocol-powering-the-future-of-connectivity/